SPECIAL MENU: Articles Index | Research Search Engines | References
Last Updated: 02.04.2020
Coronaviruses are responsible for one-third of the common colds and respiratory illnesses (ranging from mild to severe) among humans like upper respiratory tract infections (URTIs) and lower respiratory tract infections (LRTIs bronchitis, pneumonia, severe acute respiratory syndrome) (Burrell, Howard, & Murphy, 2016; Li et al., 2019; Shoeman & Fielding, 2019). These viruses are higly adaptable and prone to mutation (selective pressure, environment).
Coronaviruses belong to the order Nidovirales and family Coronaviridae. There are two subfamiles: Coronavirinae (spherical) and Torovirinae (disc, bean or rod-shaped and pertain mostly to horses, cattle, pigs, cats, and goats illnesses). Coronaviruses get their name from their unique from their “fringe-like” envelope with glycoproteins sticking out looking like a crown or crown-of-thorns (Burrell et al., 2016; Shoeman & Fielding, 2019).
Coronavirinae are subdivided into four genus categories: alpha, beta, gamma (whales, birds), & delta (pigs & birds). Coronaviruses in the alpha and beta categories viruses that have affected humans are HCoV-229E, HCoV-NL63, HCoV-OC43, SARS-HCoV, HCoV-HKU1, and MERS-CoV (Burrell et al., 2016; Shoeman & Fielding, 2019). See Table 1. SARS, SARS-like, and MERS-CoV share a good percentage of their genome with bat coronaviruses (Burrell et al., 2016; Shoeman & Fielding, 2019).
Table 1
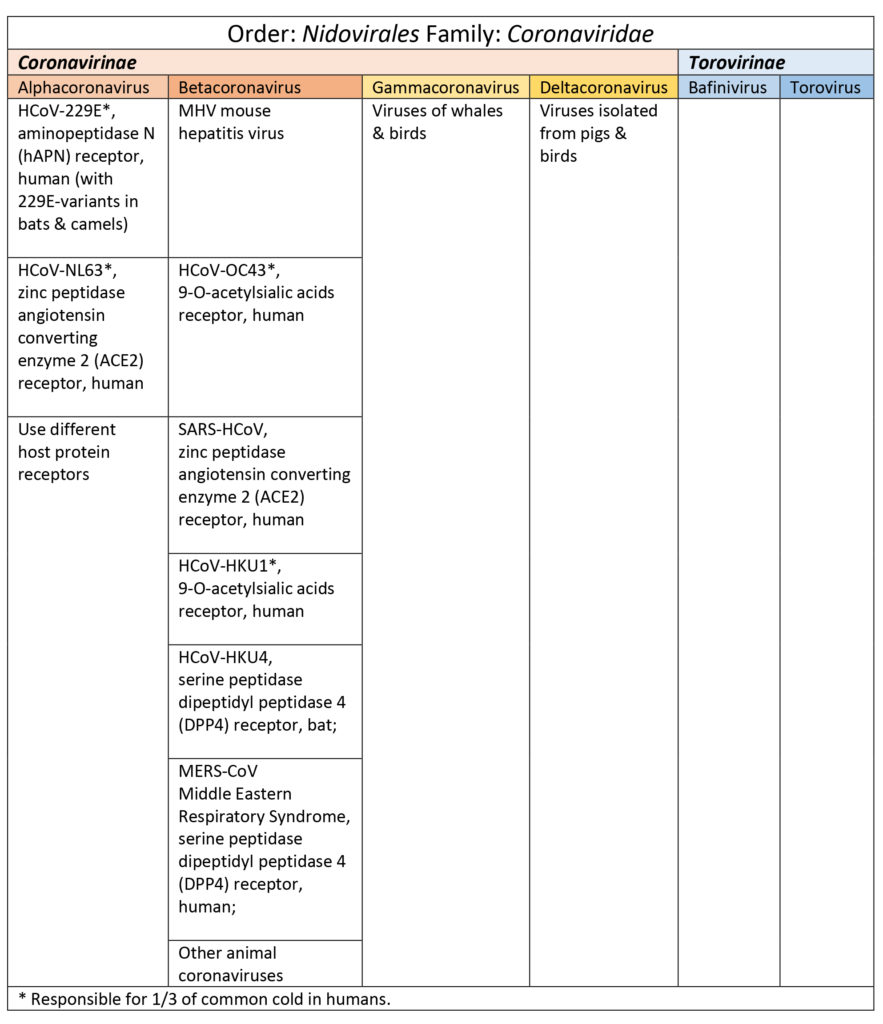
Structural Proteins
Coronaviruses have 4 key structural proteins S, M, N, and E. S (spike) protein is a large glycoprotein responsible for forming the peplomers (glycoprotein spikes which will selectively bind to certain receptors) of the envelope. M is a transmembrane glycoprotein. N is a phosphorylated nucleocapsid. Additionally, E is a envelope protein and some species of betacoronaviruses also have protein HE (hemagglutination and esterase functions) (Burrell et al., 2016; Shoeman & Fielding, 2019; Tok & Tatar, 2017). Interestingly, not all four key structural proteins are required for producing a viable virion which suggests there may be some redundancy in the genome (Shoeman & Fielding, 2019). The S, M, N, and E proteins also participate in viral replication.
Viral Genome
Coronaviruses have a single strand positive-sense RNA (+ssRNA) 26-32 kilobases (kb) long (Burrell et al., 2016; Shoeman & Fielding, 2019). This +ssRNA is of the same sense as host mRNA which allows the virus to harness the host cell’s machinery. The 5′ end (has untranslated sequence, UTR, of 65-78 nucleotides or leader RNA) is capped and the 3′ end has 200-300 UTR nucleotide sequence plus the poly-A tail (Burrell et al., 2016; Shoeman & Fielding, 2019). The 5′ cap is a way for the virus to disguise its genetic material to make it similar to host mRNA in order to use the host’s “machinery” (Picard-Jean, 2013).Nested mRNAs all share the same 5′ end sequence (Burrell et al., 2016; Shoeman & Fielding, 2019). Untranslated regions (UTRs) help regulate replication and transcription.
There are 7-14 open reading frames (ORFs) which are a sequence of codons that begin with a start codon and end with a stop codon (Burrell et al., 2016). These ORFs are interspersed within the genome.
Starting at the 5′ end (plus UTR), gene 1 (20-22 kilobases long) is about two-thirds of the entire RNA length. The spike (S) protein gene comes next followed by the E (envelope) protein gene, M (transmembrane) protein gene, the N (nucleocapsid) gene, another UTR sequence plus poly-A tail at the 3′ end (Burrell et al., 2016; Li, 2016; Shoeman & Fielding, 2019). Other ORF proteins, the HE glycoprotein gene, and accessory protein genes are interspersed amongst the aforementioned major segments (Burrell et al., 2016; Shoeman & Fielding, 2019; Tok & Tatar, 2017). Burrell et al. (2016) noted that ORFs may code for accessory proteins that may account for variability and novel species. The SARS-CoV RNA contains smaller ORFs at the 3′ end that are unique to SARS-CoV (Burrell et al., 2016). Accessory proteins may also contribute to viral hardiness.
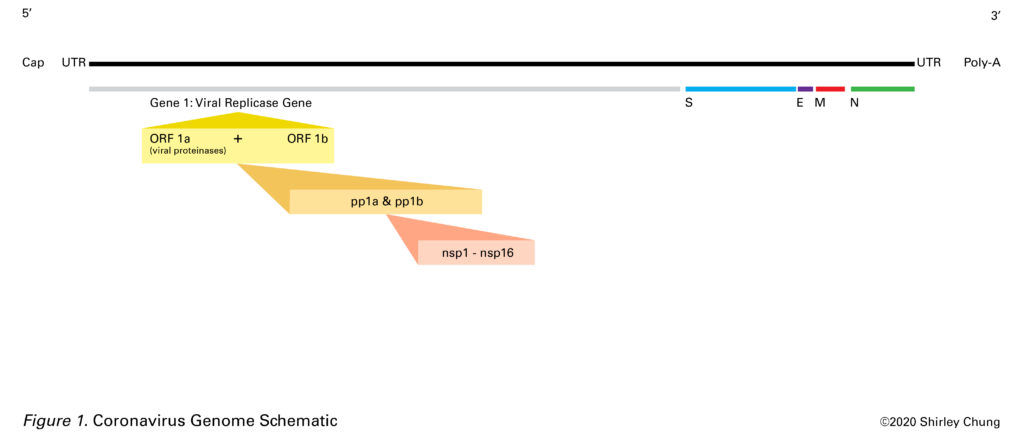
The Viral Replicase Gene (aka Gene 1)
Gene 1 contains overlapping ORFs 1a and 1b (Burrell et al., 2016). Together, ORFs 1a and 1b constitute the viral replicase gene (gene 1) and encode for two large polyproteins (pp1a and pp1b, proteins that are covalently conjoined) (Nakagawa, Lokugamage, & Makino, 2016). In general, replicase is an enzyme that catalyzes the production of a complementary RNA strand based off of an RNA template. Proteases help cleave out 15-16 nonstructural proteins (nsp1-nsp16) from the two polyproteins to be used in the viral lifecycle (Nakagawa et al., 2016). Nsp 2-nsp16 are critical to viral RNA transcription and replication (Nakagawa et al., 2016).
The S (Spike) Protein
The S glycoprotein is important as it plays a major role in the first step towards creating viral progeny. The spike determines if a virus can fuse with a host and enter. Successful fusion is receptor-dependent. S is a clove-shaped homotrimer (protein made of 3 identical subunits) (Li, 2016). The S protein is also responsible for possible multiple cell fusions between the infected and adjacent cells, syncytia (Shoeman & Fielding, 2019).
There are three classes of fusion proteins based on the structure of their fusion subunits: class I, mostly alpha-helical; class II mostly beta-structures; class III both alpha-helical and beta-structures (Harrison, 2012; White & Whittaker, 2016). Coronaviruses S protein is class I (White & Whittaker, 2016). Other class I viruses include some forms of influenza, human immunodeficiency virus (HIV), and Ebola (Li, 2017).
The spike has regions: large ectodomain (the part that sticks out into the extracellular space where upon contact may initiate signal transduction); single-pass (crosses membrane only once) transmembrane anchor (TM1); and intracellular tail (Li, 2016). The ectodomain contains S1 (trimeric head) and S2 (trimeric stalk) (Li, 2017).
For many coronaviruses, host cell enzymes (proteases) break down S into subunits S1 and S2 (Tortorici et al., 2019). Before fusion occurs, S1 and S2 are non-covalently held together. The N-terminal S1 subunit is made of four areas A, B, C, and D. A and B are the receptor-binding areas in coronaviruses (Tortorici et al., 2019). The C-terminal S2 subunit is where the “fusion machinery” is located allowing for S2 to fuse the viral and host membranes (Li, 2016; Tortorici et al., 2019). Upon entry into the host, S2 is cleaved again at S2′ (this happens for all coronaviruses) and scientists think it may be a step that activates the protein for membrane fusion as it produces irreversible conformational changes (Tortorici et al., 2019).
Post-fusion, the pre-fusion three heads of S2 become a six-bundled helical structure with fusion peptides sticking out (Li, 2017).
The N (Nucleocapsid) Protein
N is the only protein responsible for phosphorylated helical nucleocapsid (“shell” enclosing the genome) (Shoeman & Fielding, 2019). The N protein is located near the endoplasmic reticulum (ER) and Golgi complex area. Expression of the N gene was found to coincide with the increased production of virus-like particles (VLPs), and scientists believe that N may contribute towards producing whole and viable virions (Shoeman & Fielding, 2019).
The M (Transmembrane) Protein
The M glycoprotein has three transmembrane areas and influences the shape of the envelope (Shoeman & Fielding, 2019; Tok & Tater, 2017). In the Golgi complex, a carbohydrate unit is attached to the M protein (glycosylation which is important for protein folding) which contributes to the protein’s ability to bind at receptor sites (Tok & Tatar, 2017).
M may also have an “organizational” role as it interacts with the other structural proteins (Shoeman & Fielding, 2019). M seemed to interact with S protein to “corral” or aggregate S in the ER-Golgi intermediate compartment (ERGIC, mediate transport between the ER and Golgi complex) thus allowing for packaging into new virions (Shoeman & Fielding, 2019). M interacting with N increased nucleocapsid stability (Shoeman & Fielding, 2019). M and E protein interaction contributed to the maintenance of the envelope, the production and release of VLPs (Shoeman & Fielding, 2019).
The E (Envelope) Protein
E proteins (short integral membrane proteins 76-109 amino acids long) are the smallest structural proteins and the least understood. At the hydrophilic N-terminal, E has 7-12 amino acids followed by a transmembrane domain (TMD) 25 amino acids long, and ending with a long hydrophilic C-terminal (Shoeman & Fielding). Surprisingly, E protein was not found to be abundant embedded in the viral envelope which suggested that E may serve in other capacities around the ER, Golgi, and ERGIC locations (Shoeman & Fielding, 2019). E proliferation was found inside the host (near ER, Golgi complex, ERGIC) during viral replication, and the lack of E protein seemed to have deleterious effects on viral progeny (Shoeman & Fielding, 2019).
An unique feature of coronaviruses is that they bud into the ERGIC where they get packaged into their envelope (Shoeman & Fielding, 2019). In contrast, other viruses bud only from the inside face of the plasma membrane. E protein was found to play a role in scission to complete the release of viral progeny.
Transmembrane areas (TMDs) are important for the viral ability to fuse with a host (Webb, Smith, Fried, & Dutch, 2018). Viroporins are a type of transmembrane protein. Viroporins are small viral pH-sensitive pore-inducing proteins (60-120 amino acids long, mostly hydrophobic) that can create passageways (hydrophilic pores selective for H+, K+, Na+, Ca2+ but also able to transport anions) for the virus to utilize during different reproductive activities of its life cycle (Schoeman & Fielding, 2019). The lack of viroporins was found to mediate viral pathogenicity. Targeting a virus’s viroporin may be one way to interrupt it’s activities.
The HE (Hemagglutination and Esterase) Protein
The HE protein (found in some betacoronaviruses) is associated with the viral envelope. HE acts like hemagglutinin (HA) and binds to sialic acid (Sia) which is a derivative of neuraminic acids, 9-carbon backbone acidic sugars (N-acetylneuraminic acid) (Chemspider, n.d.; Langereis, Zeng, Heesters, Huizinga, & De Groot, 2012; Mora-Díaz, Piñeyro, Houston, Zimmerman, & Giménez-Lirola, 2019; Zanin, Baviskar, Webster, & Webby, 2016). Sia is sometimes used synonymously as N-acetylneuraminic acid. Sia is important in maintaining a mucous defense. HA binds to Sia of mucins and disrupts this mucous defense system (Mora-Díaz et al., 2019; Zanin et al., 2016).
Clinical
Coronaviruses favor and tend to first replicate in the respiratory or intestinal tracts (Burrell et al., 2016). They may be stable for some time outside of the human body on environmental/object surfaces (fomites) (Burrell et al., 2016). Coronaviruses may also be found in stools. Mouse hepatitis virus (MHV) is a type of betacoronavirus that can be found not only in the respiratory/intestinal tracts but also causing liver and central nervous system infections (Burrell et al., 2016). The neurological effects of MHV have been studied extensively due to its similarity to multiple sclerosis (MS) in humans (Burrell et al., 2016). Cross-immunity between different coronaviruses does not seem likely (Burrell et al., 2016). Coronaviruses are difficult to detect as an infected person may remain asymptomatic. One challenge in studying coronaviruses is that they are difficult to grow in vitro.
Closing
In order to take a step forward to try understand 2019-nCoV, it was necessary to take a step backward and try understand some of the general features of the coronaviruses. Moving forward, the next step is to learn about some of the unique features of 2019-nCoV. Undoubtedly, such knowledge at this nascent stage of viral outbreak remains fluid and dynamic.
References
Burrell, C. J., Howard, C. R., & Murphy, F. A. (2016). Fenner and White’s Medical Virology, 5.
Chemspider. (n.d.). N-Acetylneuraminic acid | C11H19NO9. Retrieved February 4, 2020, from https://www.chemspider.com/Chemical-Structure.10292217.html
Harrison, S., & Harvard Howard Hughes Medical Institute (HHMI). (2012a, March 24). Part 2: Viral membrane fusion [Video]. Retrieved from https://youtu.be/qcepGvFUM38
Harrison, S., & Harvard Howard Hughes Medical Institute (HHMI). (2013b, November 1). Class 1 viral fusion proteins [Video]. Retrieved from https://youtu.be/FcX9mD8rvYw
Langereis, M. A., Zeng, Q., Heesters, B., Huizinga, E. G., & De Groot, R. J. (2012). The murine coronavirus hemagglutinin-esterase receptor-binding site: a major shift in ligand specificity through modest changes in architecture. PLoS pathogens, 8(1).
Li F. (2016). Structure, Function, and Evolution of Coronavirus Spike Proteins. Annual review of virology, 3(1), 237–261. doi:10.1146/annurev-virology-110615-042301
Li, Z., Tomlinson, A. C., Wong, A. H., Zhou, D., Desforges, M., Talbot, P. J., … & Rini, J. M. (2019). The human coronavirus HCoV-229E S-protein structure and receptor binding. eLife, 8.
Mora-Díaz, J. C., Piñeyro, P. E., Houston, E., Zimmerman, J., & Giménez-Lirola, L. G. (2019). Porcine Hemagglutinating Encephalomyelitis Virus: A Review. Frontiers in veterinary science, 6.
Nakagawa, K., Lokugamage, K. G., & Makino, S. (2016). Viral and cellular mRNA translation in coronavirus-infected cells. In Advances in virus research (Vol. 96, pp. 165-192). Academic Press.
National Human Genome Research Institute (NHGRI). (n.d.). Open Reading Frame. Retrieved from https://www.genome.gov/genetics-glossary/Open-Reading-Frame
Picard-Jean, F., Tremblay-Létourneau, M., Serra, E., Dimech, C., Schulz, H., Anselin, M., … & Bisaillon, M. (2013). RNA 5′-end maturation: a crucial step in the replication of viral genomes. Current Issues in Molecular Virology: Viral Genetics and Biotechnological Applications, 27.
Schoeman, D., & Fielding, B. C. (2019). Coronavirus envelope protein: current knowledge. Virology journal, 16(1), 69.
Tok, T. T., & Tatar, G. (2017). Structures and Functions of Coronavirus Proteins: Molecular Modeling of Viral Nucleoprotein.
Tortorici, M. A., Walls, A. C., Lang, Y., Wang, C., Li, Z., Koerhuis, D., … & Veesler, D. (2019). Structural basis for human coronavirus attachment to sialic acid receptors. Nature structural & molecular biology, 26(6), 481-489.
University of Washington Health Sciences/UW Medicine. (2016, February 26). Electron microscopy captures snapshot of structure coronaviruses use to enter cells: Atomic model suggests vaccine strategies against deadly pandemic viruses such as SARS-CoV and MERS-CoV. ScienceDaily. Retrieved January 31, 2020 from www.sciencedaily.com/releases/2016/02/160226173209.htm
Webb, S. R., Smith, S. E., Fried, M. G., & Dutch, R. E. (2018). Transmembrane Domains of Highly Pathogenic Viral Fusion Proteins Exhibit Trimeric Association In Vitro. mSphere, 3(2), e00047-18.
White, J. M., & Whittaker, G. R. (2016). Fusion of enveloped viruses in endosomes. Traffic, 17(6), 593-614.
Zanin, M., Baviskar, P., Webster, R., & Webby, R. (2016). The interaction between respiratory pathogens and mucus. Cell host & microbe, 19(2), 159-168.