Last edited: 08.14.2019
Outline.
- Tricarboxylic Acid Cycle (TCA Cycle).
- Electron Transport Chain (ETC) and Oxidative Phosphorylation.
- Glycolysis and Gluconeogenesis.
TCA Cycle.
Net Equation.
3NAD+ + FAD + FDP + Pi + Acetyl-CoA + 2H2O >>> 3NADH + FAD(2H) + GTP + CoASH + 2CO2
- Metabolism: sum total of all chemical reactions in a cell.
- Anabolism: building up; synthesis (especially regarding energy and building materials).
- Catabolism: breaking down (especially for energy).
- Tricarboxylic Acid Cycle (TCA) = Citric Acid Cycle = Krebs Cycle
- The TCA cycle is responsible for more than 2/3 of ATP production from fuel oxidation.
- The conversion of pyruvate into acetyl-CoA takes place inside the mitochondria.
- TCA occurs in the matrix of mitochondria (eukaryotes).
- Biochemical pathways are different routes that reactants, intermediates, products and etc. can take to lead to different outcomes in order to produce what is needed by the cell/organism. This builds on the concept of basic building blocks make more complex molecules that is needed, and then breaking down the complex molecules back to basic units in ordered to be recycled. Concept of “shuttling”. Intermediates may be recycled-reused and/or may be important to the biosynthesis of other biomolecules.
- One of the important goals for the oxidation of FAs, glucose, AAs, acetates and ketones is to convert these materials into acetyl coenzyme A (Acetyl CoA) which is the form that is needed in order to enter the TCA cycle.
- Goals is to conserve energy from all the oxidation reactions.
- Electrons from intermediates get transferred to NAD+ and FAD.
- 8 electrons given up from the acetyl group end up in 3 molecules of NADH and 1 FADH2.
- This is important because the carriers NADH and FADH2 give the electrons to oxygen as the final electron acceptor in the electron transport chain (ETC).
- Good nutrition plays an important role. This is what the TCA needs: lots of vitamin and minerals; niacin (NAD+); riboflavin (FAD) and flavinmononucleotide; pantothenic acid (coenZ A); thiamin; Mg 2+, Ca 2+, Fe 2+, phosphate.
- Sources of Acetyl-CoA include (but not limited to): beta-oxidation of fatty acids such as palmitate; breakdown of ketone bodies beta-hydroxybutyrate and acetoacetate; acetate (from diet or ethanol oxidation); glucose/carbohydrates oxidized to pyruvate; alanine and serine can also be oxidized to pyruvate.
- Regulatory steps tend NOT to be reversible (with the exception of step 8, malate <–> oxaloacetate).
Quick Redox Review.
- LEO, Lose Electrons Oxidation. Gain oxygen or lose hydrogen.
- GER, Gain Electrons Reduction. Lose oxygen or gain hydrogen.
- *The more multiple bonds, the greater degree of oxidation.
- In biochem, it’s not as “obvious” to see how the electrons flow and what is oxidized and what is reduced.
- Sometimes it helps to look at the number of bonds and how the bonds change.
- If A is losing electrons/bonds, then it is getting oxidized (it is being oxidized).
A’s role is the reducing agent. - If B is gaining electrons/bonds, then it is being reduced.
B’s role is the oxidizing agent.
Acetyl CoA.
- Examining Acetyl CoA, 2 main structural parts are easily recognizable: phosphopantetheine chain and nucleotide.
- The phosphopantetheine chain has -SH thiol group (can form thioesters with acyl groups) at the end of the pantothenic acid.
- The nucleotide is adenosine 3′, 5′ -biphosphate.
- The CoA-S-(C=O)-R helps to activate fatty acid or acetyl group.
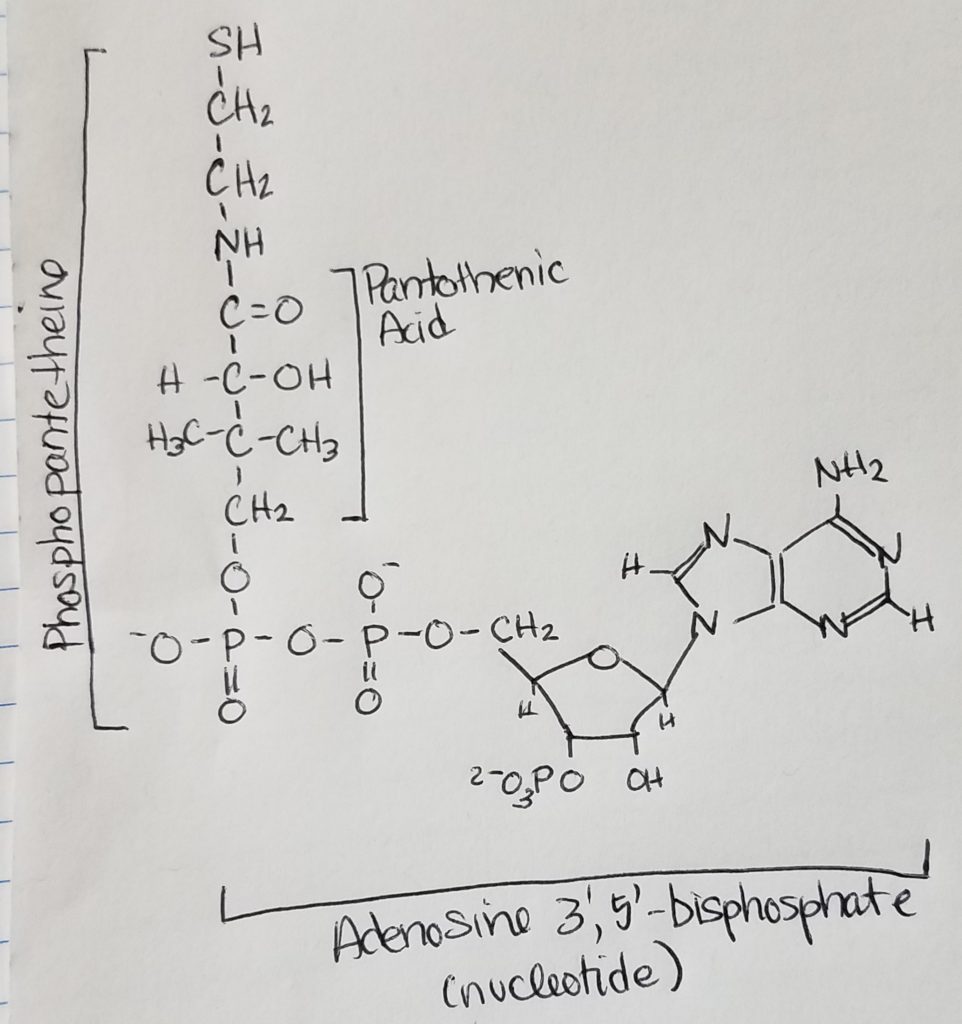
Pyruvate Dehydrogenase Complex (PDHC/PDC).
- From the alpha-ketoacid dehydrogenase complex family.
- 3 catalytic subunits:
1. Pyruvate decarboxylase subunits (bind TPP E1)
2. Transacetylase subunits bind lipoate (E2)
3. Dihydrolipoyl dehydrogenase subunits that bind FAD (E3) - PDHC has 2 regulatory enzymes: pyruvate dehydrogenase kinase (PDH kinase) and pyruvate dehydrogenase phosphatase (PDH phosphatase).
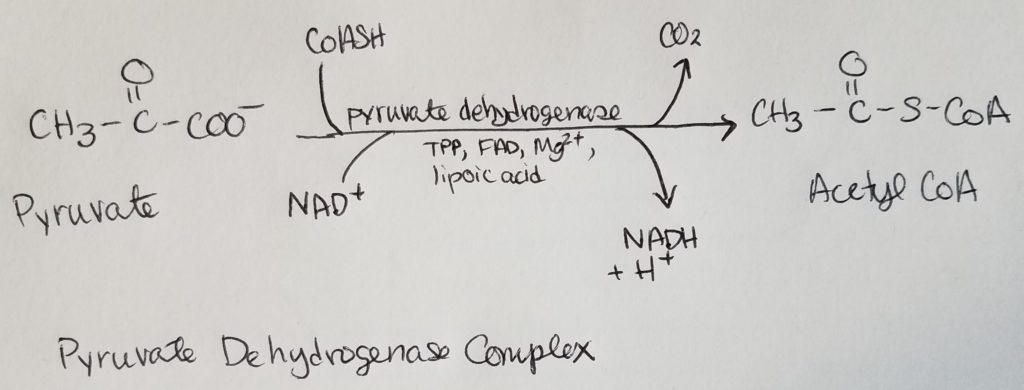
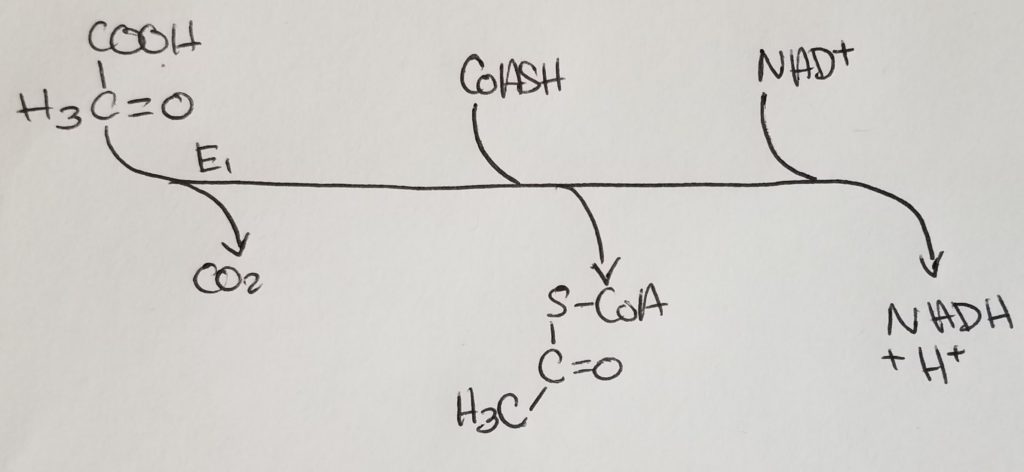
STEP 1. Citrate Synthase Rxn.
- Acetyl-CoA + oxaloacetate + H2O >>citrate synthase>> Citrate (6 carbons) + CoA-SH
- Reactants: acetyl-CoA, oxaloacetate, water.
- Enzyme: citrate synthase.
- Products: citrate.
- Leaving: CoASH.
- Irreversible reaction.
- Regulation: -citrate.
- “Synthases” generally work by catalyzing the condensation reaction of 2 organic molecules to form a C-C bond when no high-energy phosphate bond energy is available.
- “Synthetase” (note the spelling looks a lot like “synthase”) are enzymes that DO need the high-energy phosphate bond energy to do their work.
- *Oxaloacetate is regenerated w/each turn of this cycle.
- The condensation of Acetyl-CoA and oxaloacetate (OAA) is irreversible .
- Acetyl-CoA can come from: palmitate, acetoacetate, glucose, pyruvate, alanine, and ethanol (PPAAGE). However, the major source is oxidative decarboxylation of pyruvate via pyruvate dehydrogenase complex (PDHC).
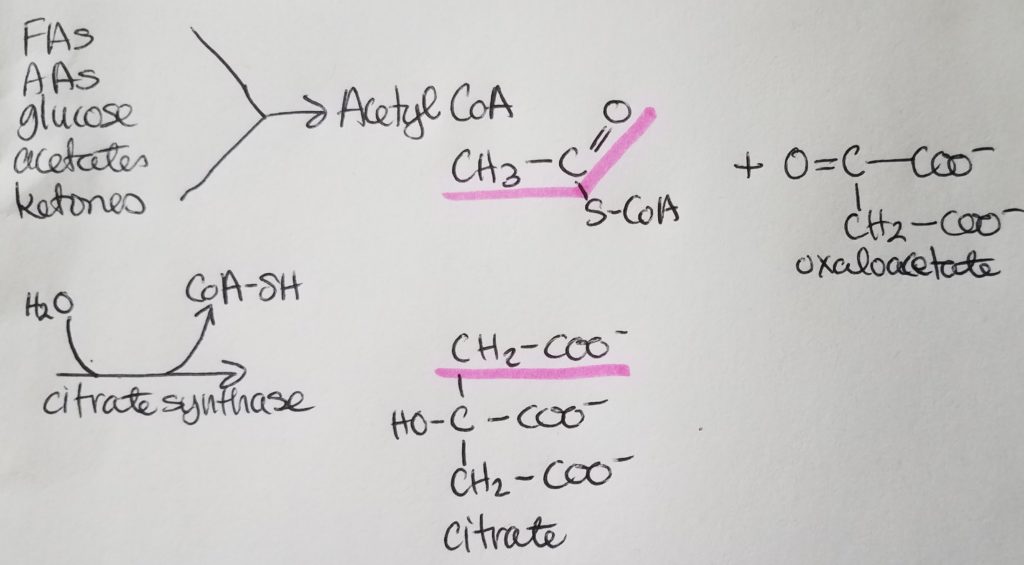
STEP 2. Isomerization of Citrate.
- Citrate (a tricarboxylate acid) is rearranged to isocitrate.
- Reactants: citrate.
- Enzyme aconitase (Fe-S protein), isocitrate dehydrogenase.
- Intermediate: cis-aconitate.
- Product: isocitrate.
- Reversible reaction.
- Aconitase catalyzes the migration of -OH group to a neighboring carbon so that it can be oxidized to form part of a keto-group.
- Aconitase is inhibited by a pesticide/plant toxin called fluoroacetate. Fluoroacetate gets converted to fluorocitrate which is an inhibitor.
- Isocitrate dehydrogenase catalyzes the oxidation of the -OH and cleaves the carboxyl, releaseing CO2.
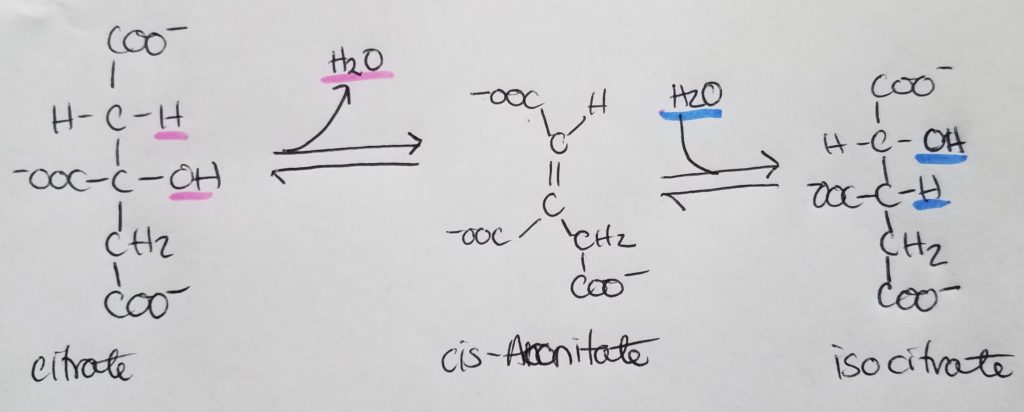
STEP 3. Isocitrate Dehydrogenase Rxn.
- Oxidative decarboxylations: these are oxidations reactions (Lose Electrons Oxidation) where a carboxylate is removed and CO2 is produced.
- Isocitrate undergoes oxidative decarboxylation (releasing CO2) to form alpha-ketoglutarate.
- Reactants: Isocitrate, NAD+.
- Enzyme: isocitrate dehydrogenase.
- Products: alpha-ketoglutarate, CO2, NADH + H+.
- Irreversible reaction.
- Regulation: -NADH, +ADP, +Ca+2.
- One NAD+ is reduced to NADH.
- This is the first NADH produced in the TCA cycle.
- Isocitrate + NAD+ >>isocitrate dehydrogenase>> Oxalosuccinate + NADH + H+
- This is a rate-limiting step as isocitrate dehydrogenase is allosterically activated by ADP and Ca+2 and inhibited by ATP and NADH.
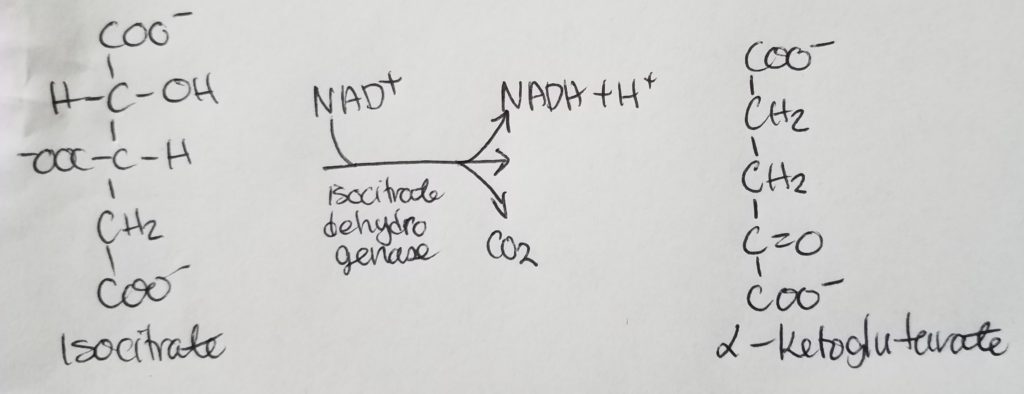
STEP 4. Oxidation of Alpha-Ketoglutarate.
- Alpha-ketoglutarate undergoes oxidative decarboxylation to form succinyl-CoA.
- Reactants: alpha-ketoglutarate, CoASH.
- Enzymes: alpha-ketoglutarate dehydrogenase complex, protein aggregate (contains conenzymes TPP or thiamine pyrophosphate, lipoic acid, FAD).
- Products: succinyl CoA, CO2, NADH + H+.
- Irreversible reaction.
- Regulation: -NADH, +Ca+2.
- CO2 is released from one of the carboxyl groups of alpha-ketoglutarate.
- Second NADH is released.
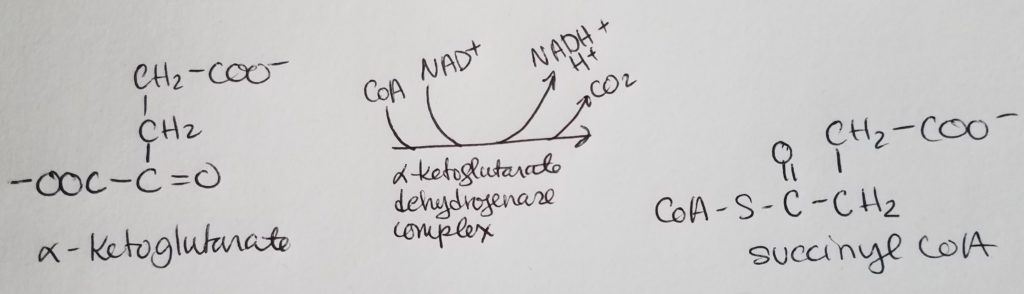
STEP 5. Formation of Succinate.
- Succinyl-CoA >> succinate thiokinase >> Succinate
- Reactants: succinyl CoA, GDP + Pi.
- Enzyme: succinate thiokinase (cleaves thioester bond and the bond energy is used to make GTP from GDP and Pi).
- Products: succinate, GTP, CoASH.
- Reversible reaction.
- Note that guanosine diphosphate (GDP) gets phosphorylated to GTP.
GTP and ATP are interconvertible via nucleoside diphosphate kinase:
GTP + ADP <–> GDP + ATP - Substrate-level phosphorylation: forming a high-energy phosphate bond (with none having preexisted) from molecular O2 and NOT from oxidative phosphorylation.
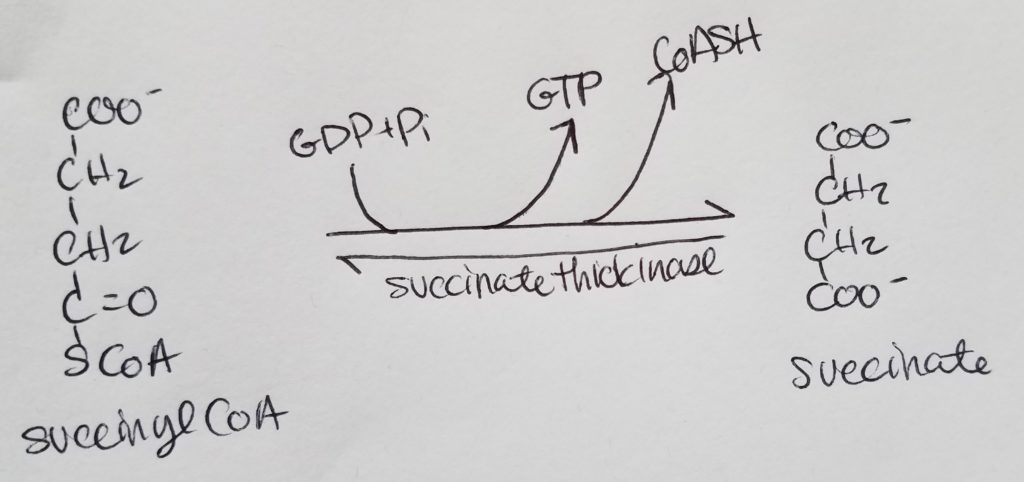
STEP 6. Formation of Fumarate.
- Succinate >>succinate dehydrogenase>> Fumarate
- Reactants: succinate, FAD.
- Enzyme: succinate dehydrogenase.
- Products: fumarate, FADH2.
- Reversible reaction.
- The coenzyme FAD gets reduced to FADH2.
- Succinate dehydrogenase is the only enzyme in the TCA that is in the inner mitochondrial membrane.
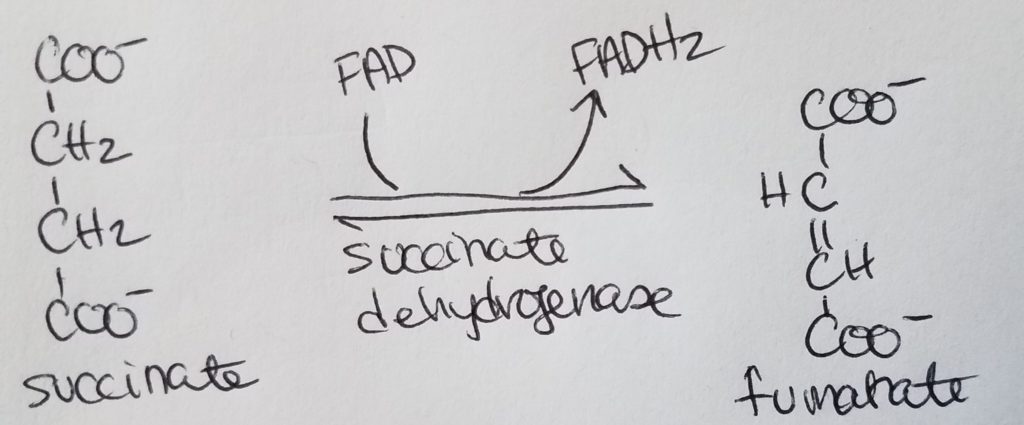
STEP 7. Formation of Malate.
- Fumarate >>fumarate hydratase>> Malate
- Reactants: fumarate, water.
- Enzyme: fumarase.
- Product: Malate.
- Reversible reaction.
- This is a hydration reaction.
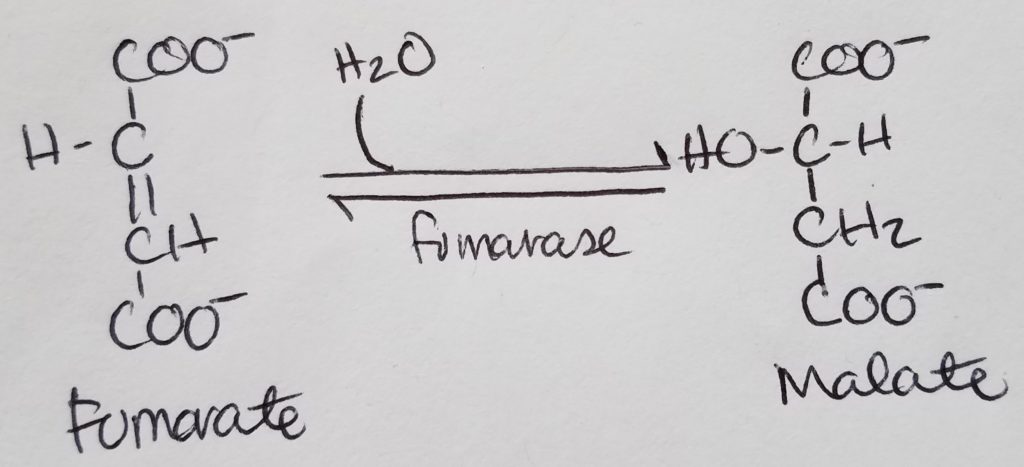
STEP 8. Formation of Oxaloacetate.
- Malate >>malate dehydrogenase>> Oxaloacetate
- Reactants: malate, NAD+.
- Enzyme: malate dehydrogenase.
- Products: oxaloacetate + NADH + H.
- Reversible reaction AND a regulatory step.
- Regulation: -NADH.
- This step makes the third NADH.
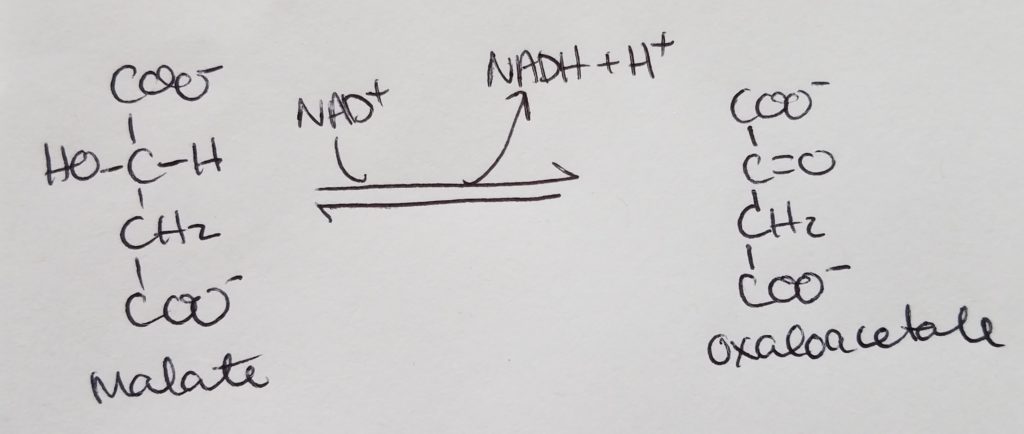
Summary of TCA Step 1-8.
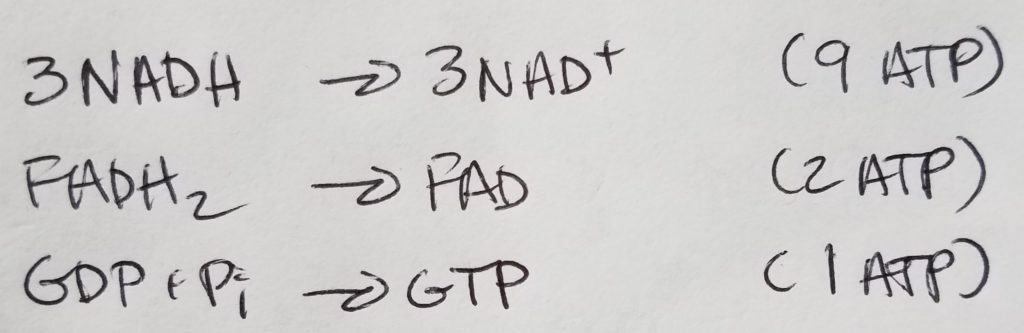
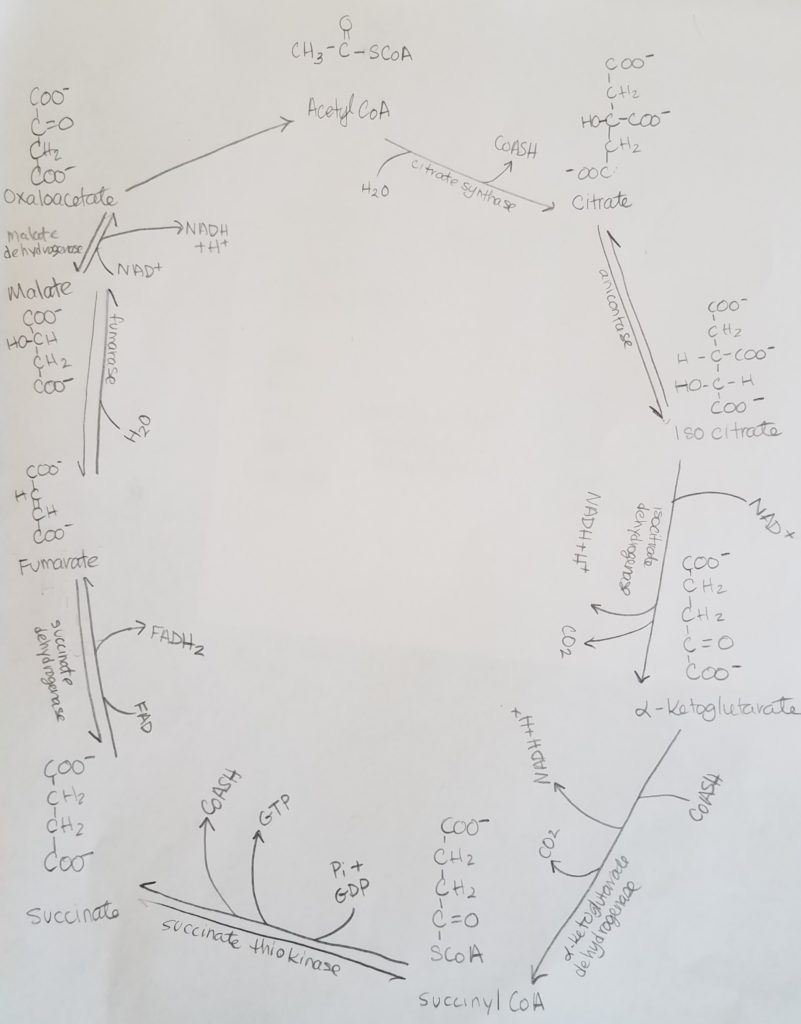
TCA Regulation.
- The rate of TCA is regulated to correspond to the rate of ETC.
- ETC is regulated by the ratio of ATP:ADP and the rate of ATP usage.
- ATP Rate of Usage Feedback:
1. phosphorylation state of ATP (ratio of ATP:ADP)
2. reduction state of NAD+ (ratio of NADH:NAD+) - In the cell and also in mitochondria, the pools of total adenine and the pools of total NAD are fairly/relatively constant.
Citrate Synthase Regulation.
- Has no allosteric regulators.
- Rate is controlled by concentration of oxaloacetate and the concentration of citrate (product inhibitor and competitor of oxaloacetate)
- Malate-oxaloacetate equilibrium favors malate. Oxaloacetate concentration is low inside mitochondria.
- As the NADH:NAD+ ratio increases, the oxaloacetate:malate ratio also increases.
- Activation of isocitrate dehydrogenase decreases the concentration of citrate, suppressing the inhibitory effect of citrate synthase.
- Liver NADH:NAD+ ratio helps determine if Acetyl-CoA enters the TCA or another pathway for ketone production.
Isocitrate Dehydrogenase Regulation.
- Isocitrate dehydrogenase is made up of 6 subunits.
- Is one of the rate-limiting steps in TCA.
- Allosterically activated by ADP.
- Also activated by Ca+2. The release of Ca+2 from the sarcoplasmic reticulum may provide additional activation.
- Inhibited by NADH.
- When ADP is absent, the subunits bind to each other and are converted to an active conformation.
- When ADP is present, all the subunits are in active conformation enabling isocitrate to bind more easily and readily.
- A small change in the concentration of ADP can significantly affect this step.
Alpha-Ketoglutarate Dehydrogenase Regulation.
- Alpha-ketoglutarate is a complex.
- It isn’t allosterically regulated.
- It is product-inhibited (negative feedback) by NADH and succinyl-CoA (and maybe GTP as well).
- Also activated by Ca+2. The release of Ca+2 from the sarcoplasmic reticulum may provide additional activation.
TCA Intermediates Regulation.
- TCA regulation ensures that the rate of NADH generation is sufficient to maintain ATP homeostasis, AND regulates the concentration of all the intermediates.
The Electron Transport Chain (ETC) & Oxidative Phosphorylation.
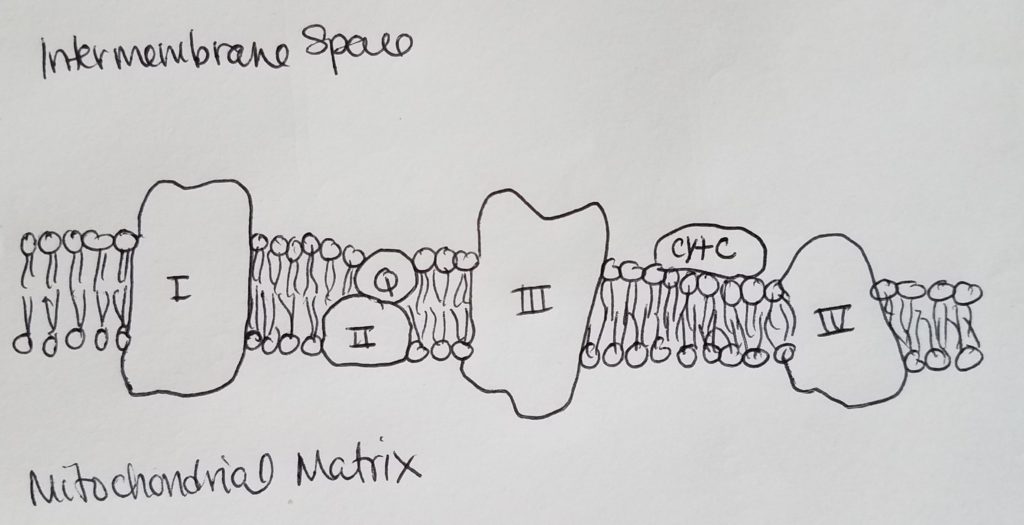
- Chemiosmotic Model of ATP Synthesis.
- ETC occurs in the inner mitochondrial membrane, the site of oxidative phosphorylation via ATP synthase.
- A series of oxidative reactions using oxygen as the final electron acceptor, release water and carbon dioxide.
- The intermediates of this oxidative series donate their electrons to coenzymes, adenine dinucleotide (NAD+), and flavin adenine dinucleotide (FAD). The reduced NADH and FADH2 have lots of energy stored in their bonds.
- As electrons are shuttled down this chain, they lose their free energy. This energy gets redirected to transport H+ (protons) from the mitochondrial matrix across the inner membrane of the mitochondria to cytosolic side thus causing an H+ gradient enabling the production of ATP from ADP + inorganic phosphate, Pi.
- This “proton pump” creates an electrochemical gradient. This is key.
- ATP synthase has a pore from the inner membrane to the headpiece sticking out in the matrix. Protons are pushed through this pore changing the conformation of the headpiece and this causes the simultaneous release of ATP at one site and the formation of ATP from ADP + Pi at another site.
Mitochondria.
- Inner mitochondrial membrane, rich in proteins, is impermeable to most small ions (including H+) and molecules like ADP, ATP, pyruvate, etc. The mitochondria need specialized carriers to transport goods inside. Cristae (convolutions) help to increase the surface area.
- The inner membrane has 4 separate complexes which are part of the ETC.
- ETC has 3 large protein complex I, III, & IV spanning the inner mitochondrial membrane.
- All members of the ETC, except CoQ (lipid-soluble quinone), are proteins.
- ATP that’s made is actively transported into the intermembrane space by adenine nucleotide translocase (ANT).
- Yields 30-32 ATP.
- Matrix maintains a low concentration of H+, high pH.
- Intermembrane space maintains a high concentration of H+ low pH.
- Proton pumps pump H+ from the matrix to the intermembrane space. Because this is against the concentration, it takes energy/work to maintain this potential gradient.
Electron Carriers.
- NAD+ (nicotinamide adenine dinucleotide). Related to vitamin B3, niacin. Indirectly produces 2.5-3 ATPs.
NADH –> NAD+ + H + 2e- - FAD (flavin adenine dinucleotide). Related to vitamin B2, riboflavin. Its electrons are at a lower energy state. Indirectly produces 1.5-2 ATPs.
- FMN (flavin mononucleotide). Vitamin B2, riboflavin. A prosthetic group, a non-protein molecule that is needed for the function of a protein.
- Other: Fe-S complexes, cytochromes, copper-based carriers.
- 2e- + H+ + 1/2O2 = water (this is the reduction of oxygen to water, or the oxidation of NADH to NAD+)
- Ubiquinone (Q) carries electrons from complex I and complex II to complex III. It is lipid-soluble and moves in the hydrophobic core of the membrane. Q carries pairs of electrons (2e-).
- Cytochrome C. Carries electrons from complex III to complex IV. Cytochrome C can carry only one e- at a time.
- Cytochrome B.
- Cytochrome A.
- Cytochrome A3.
Complex I and Q.
- NADH carries 2e- to complex I.
- Complex I is the entrypoint for NADH.
- Complex I is made of FMN (vitamin B2) and Fe-S.
- NADH dehydrogenase is the enzyme in complex I.
- Complex I pumps 4 H+ into the intermembrane space.
- Ubiquinone (Q) takes the 2e- and shuttles them to cytochrome C which shuttles them to complex III.
Complex II (succinate dehydrogenase).
- Complex II is the entry point for FADH2.
- FADH2 ‘s electrons are dropped off and Q shuttles them to complex III.
- Q, a mobile electron carrier like a “taxi”, is reduced to QH2.
- Complex II is bound to the membrane.
- Complex II is not a pump.
Complex III (cytochrome oxidoreductase).
- Complex III is composed of: Fe-S, cytochrome b, Reiske centers (2Fe-2S), cytochrome c1.
- Complex III pumps 4 H+ to the intermembrane space.
- The electrons get passed to complex IV via cytochrome c. Cytochrome c can carry only one e- at a time (not 2e-).
- Electrons get passed from cytochrome b to Fe-S to cytochrome c1.
Complex IV.
- Complex IV is made of cytochromes c, a, and a3.
- Complex IV has 2 heme groups per cytochromes a and a3. An oxygen molecule is bound very tightly between the iron and copper ions until the oxygen can be totally reduced at which point it picks up two H+ from the surrounds for the creation of water.
- 2H+ gets pumped out to the intermembrane spae.
Summary of ETC.
So thus far, the main purpose of ETC is two-fold: maintain the [H+] concentration gradient (high [H+] in the intermembrane space, and low [H+] in the matrix), and the shuttling of e- from a higher energy state to lower energy state where oxygen is the final electron acceptor and is expelled by binding it to two H+ to form water. Complexes I, III, and IV are proton pumps while complex II is like a proton funnel.
Chemiosmosis & ATP Synthase.
- Chemiosmosis is when the movement of ions down their electrochemical gradient is harnessed “to do work”. In this case, “work” is putting ADP and Pi together to form ATP.
- This process accounts for more than 80% of the ATP produced in the human body.
- ATP synthase is a proton channel (the only kind in the intermembrane space of mitochondria) allowing H+ from the intermembrane space to flow down their concentration gradient back into the matrix.
- ATP synthase is like a water-wheel or wind/water turbine. For every four H+ that flows through ATP synthase (from the intermembrane space to the matrix), one ADP + Pi bond can be “built” resulting in ATP.

Glycolysis.
- Glycolysis is the breakdown of glucose.
- This process occurs in the cytosol and doesn’t require oxygen (can work in the presence of oxygen or without oxygen).
- There are 2 stages. Stage 1 is the “spending” stage where ATP is spent. Stage 2 is the “money-making” stage where we make some “ATP money” back.
- In the larger picture, the real advantage of glycolysis is making that pyruvate, then converting the pyruvate into acetyl CoA (in the mitochondria) and entering the TCA cycle and ETC (where the most amount of ATP is made…like winning the lottery).

Stage 1. Step 1. Glucose to Glucose 6-phosphate.
- This step is a commitment. Once a phosphate groups is attached to glucose, glucose cannot leave the cell.
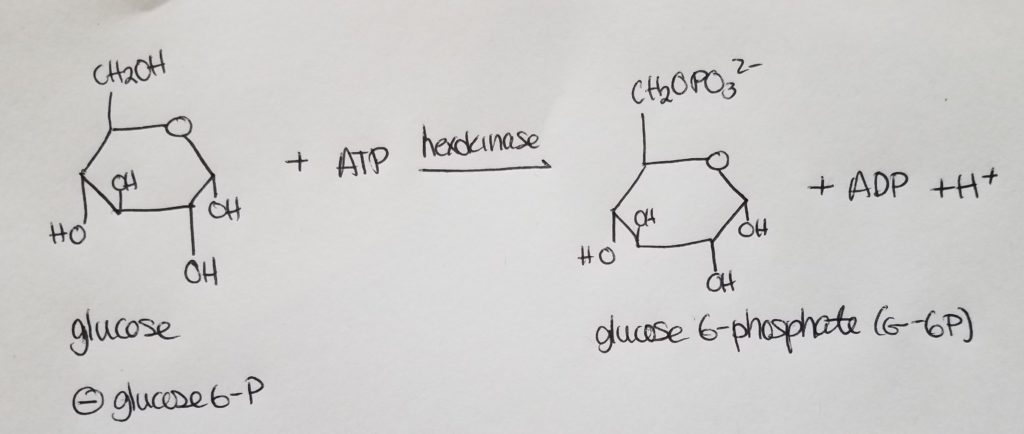
Stage 1. Step 2.
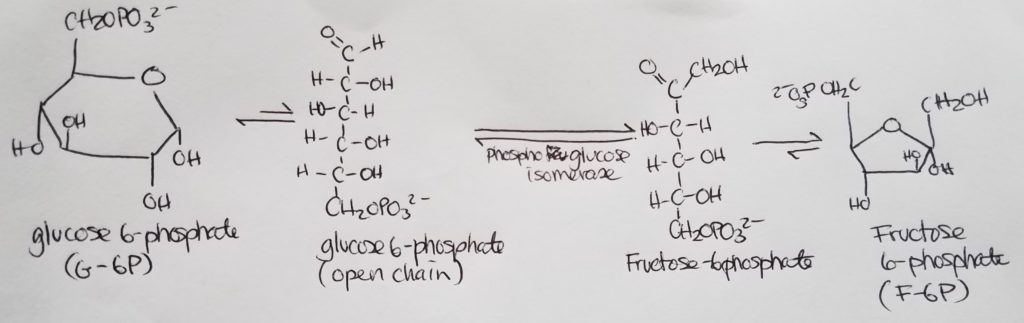
Stage 1. Step 3.
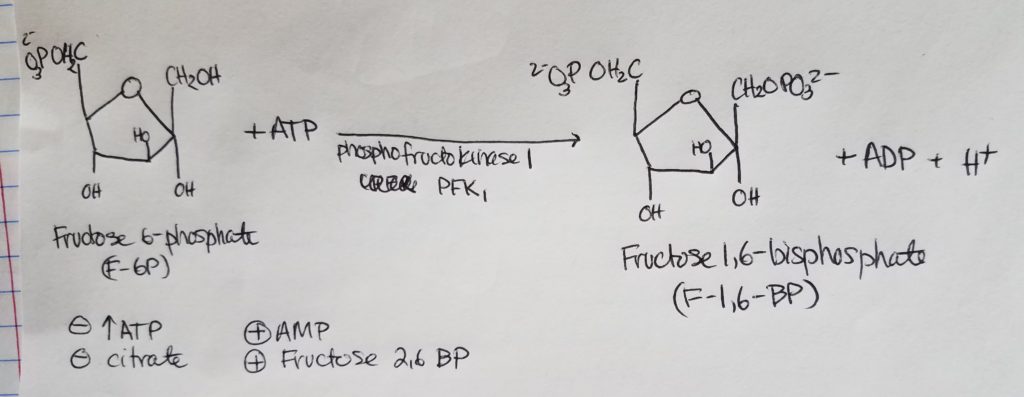
- This is the primary regulatory step for glycolysis.
- Fructose 1,6-bisphosphate is not a “real intermediate” (meaning it doesn’t act as an “intermediate”) but plays a more regulatory role in that its presence will either inhibit or promote an action.
- PFK1 is a rate-limiting enzyme via allosteric regulation.
- Inhibited by high concentrations of ATP.
- Inhibited by citrate concentrations.
- Promoted/stimulated by high concentrations of AMP (adenosine monophosphate).
- Promoted/stimulated by high concentrations of fructose 2,6-bisphosphate.
- If citrate appears in the cytosol, it can “leak out” and slow down the TCA cycle because an abundance of citrate indicates that the energy needs requirements are met–we have adequate energy/fuel. Citrate builds up in the mitochondrial matrix and “leaks” out into the cytosol.
Stage 1. Step 4.
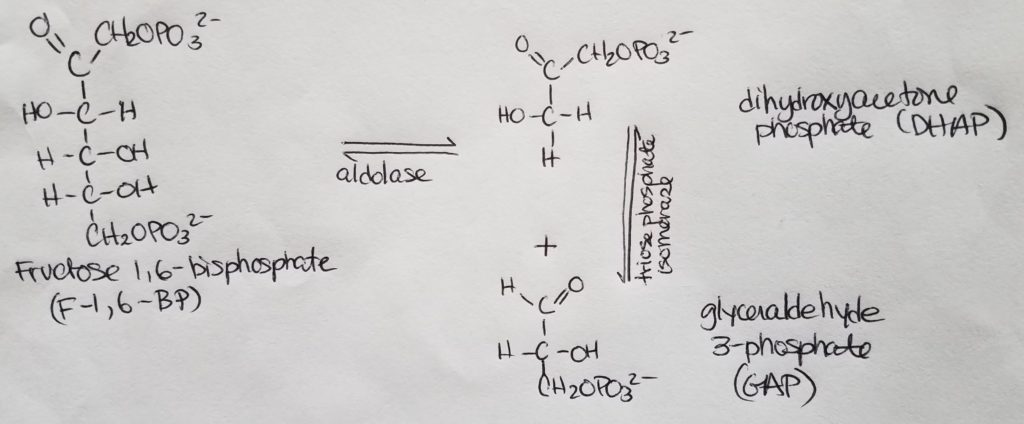
- A 6-carbon molecule splits into two 3-carbon molecules which are isomers of each other. The only form that will continue on in the glycolysis process is glyceraldehyde 3-phosphate (GAP).
- The two 3-carbon isomers “flip” configuration back-and-forth via triose phosphate isomerase. This is noted in the next step 5.
Stage 1. Step 5.
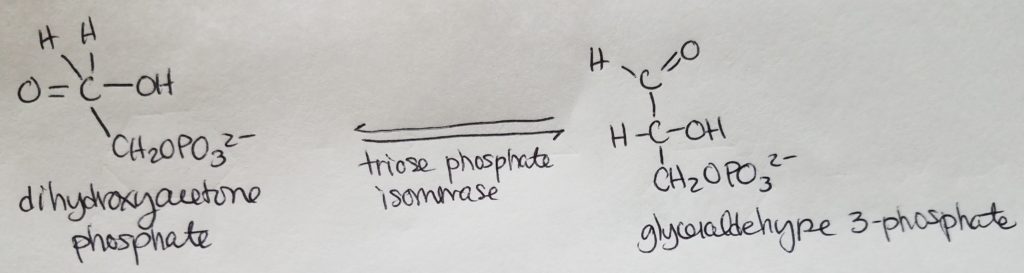
- While these two isomers coexist with equal likelihood, the only form that will continue on in the glycolysis process is glyceraldehyde 3-phosphate.
Stage 2. Step 6.
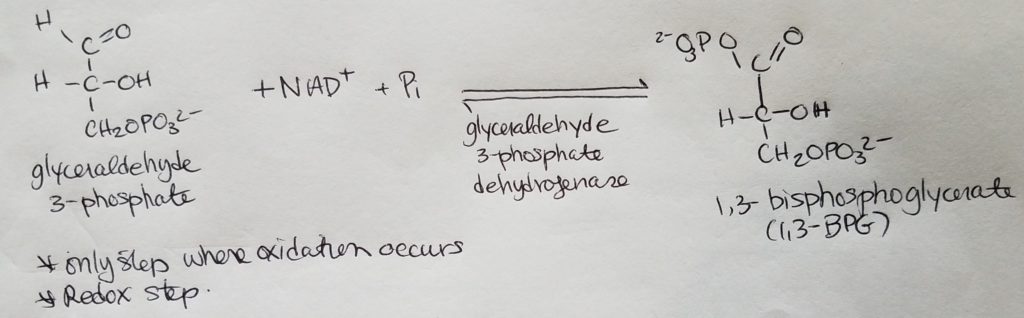
- This is the only step where oxidation occurs.
- This step is a redox reaction.
Stage 2. Step 7.
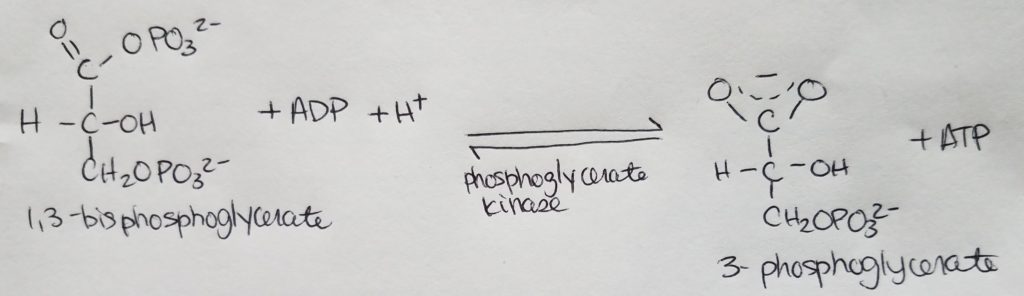
- Substrate level formation of ATP.
- Note the resonance structure of 3-phosphoglycerate.
Stage 2. Step 8.
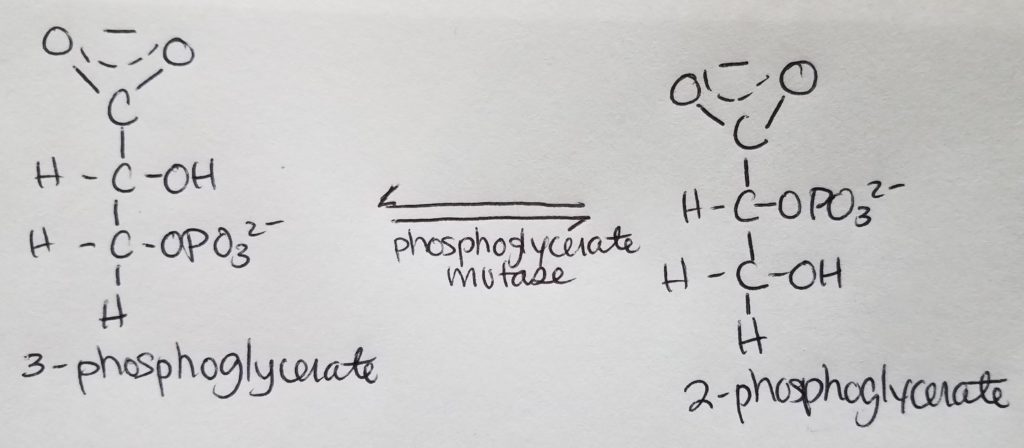
- Mutase phosphate on carbon 2.
Stage 2. Step 9.
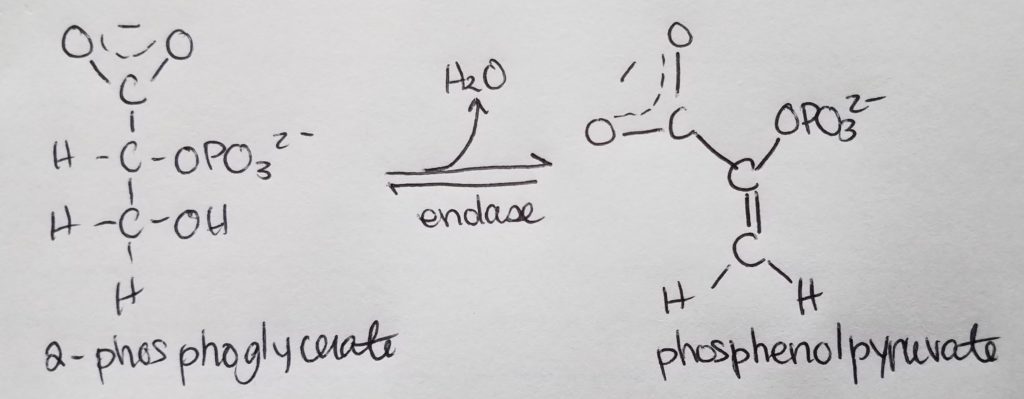
Stage 2. Step 10.
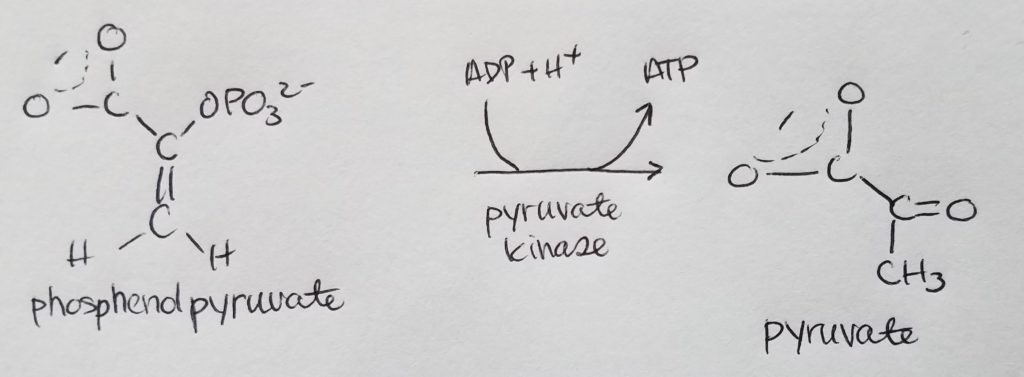
- Substrate level ATP formation.
- Inhibited by high concentration of ATP, product-inhibition.
- Promoted/activated by fructose 1,6-bisphosphate.
Recap.
- In Stage 1, two ATP was “spent” or -2 ATP.
- In Stage 2, 4 ATP were created.
- Net ATP = 2.
- In Stage 2, 2 NADH were created.
- In Stage 2, 2 molecules of water were created.
- In Stage 2, 2 molecules of pyruvate were created.
Regulation of Glycolysis.
- Glycolysis is regulated at 5 enzymatic areas (and their corresponding step): hexokinase; phosphofructokinase; glyceraldehyde 3-phosphate dehydrogenase; phosphoglycerate kinase; and pyruvate kinase.
- Hexokinase (glucose to glucose 6-phosphate): inihibited by high levels of glucose 6-phosphate (product inhibition which is a form of negative feedback).
- Phosphofructokinase (fructose 6-phosphate to fructose 1,6-bisphosphate): most important as a rate-limiting step in mammalian glycolysis; this is the first committed step which means that if we make the product for this step, we are committed to the rest of the glycolytic pathway. Fructose 1,6-bisphosphate is the product. Inhibited by: high ATP concentrations; high citrate concentrations. Activated by: high AMP concentrations; high fructose 2,6-bisphosphate (formed in the FED state when glucose is abundant). Fed/absorptive state is up to 4 hours after a meal.
- Pyruvate kinase (phosphoenolpyruvate to pyruvate): inhibited by high ATP concentrations; activated by high fructose 1,6-bisphosphate concentratoins.
Alternate Paths for Pyruvate.
- Glycolysis makes pyruvate.
- From there, pyruvate can be used in many different pathways: alcohol fermentation (anaerobic microbial/bacterial process); lactate fermentation (anaerobic); aerobic respiration (conversion of pyruvate to acetyl CoA, TCA cycle, ETC).
Alcohol Fermentation.
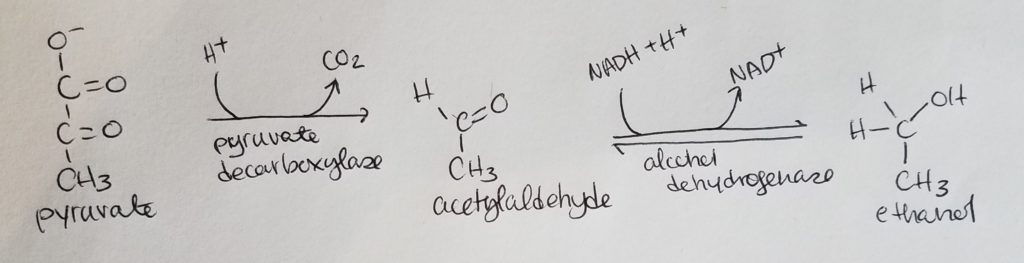
- Results in 2 ATP.
- Anaerobic pathway.
Lactate Fermentation.
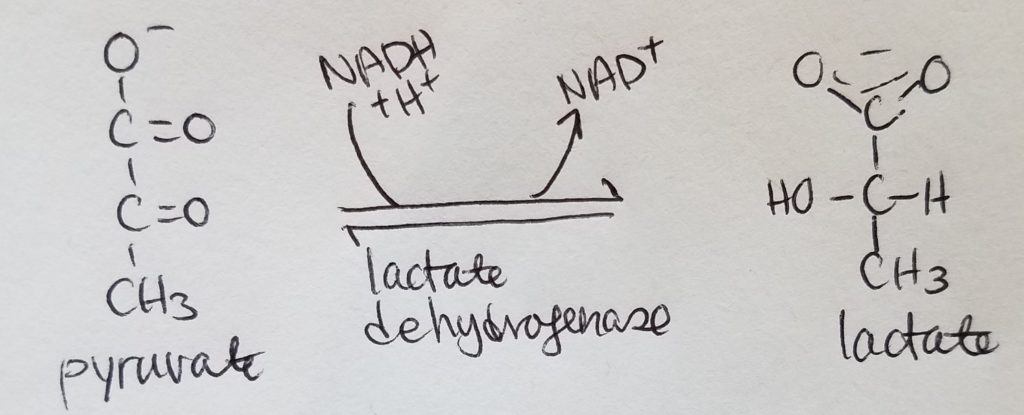
- Results in making 2 ATP.
- Anaerobic pathway.
- Occurs in muscle cells under anaerobic conditions.
Entrypoints of Other Sugars.
- Fructose (mobilized from adipose tissue) enters glycolysis at fructose 6-phosphate.
- Galactose enters glycolysis at glucose 6-phosphate.
Gluconeogenesis.
- Gluconeogenesis is the process by which pyruvate is converted to glucose; glucose is generated from non-carbohydrate sources (lactate, amino acids, fatty acids).
- If there’s an increase in glycolytic activity, gluconeogenesis is suppressed.
- If there’s a decrease in glycolytic activity, gluconeogenesis is promoted/activated.
- Gluconeogenesis is not “reverse glycolysis” because glycolysis contains 3 irreversible steps. SO, we need to find a “workaround” for those 3 irreversible steps in glycolysis.
- Gluconeogenesis is a highly aerobic pathway!
Roadblock 1. How can we get to phosphoenol pyruvate from pyruvate?
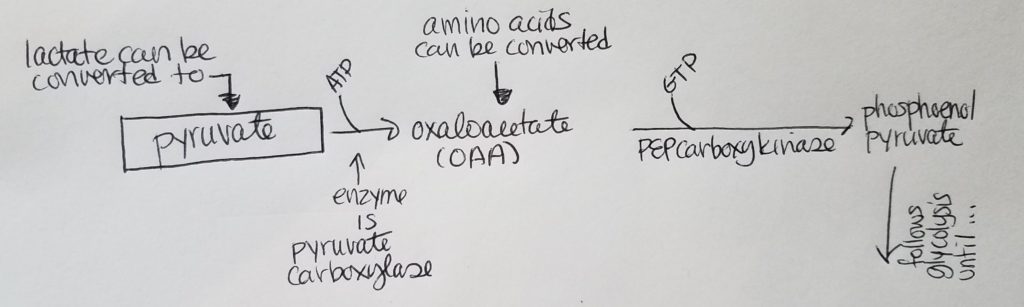
- Side note: lactate can be converted to pyruvate.
- Let’s start with pyruvate. We want to convert pyruvate to phosphoenol pyruvate so that we can reuse and follow most of the reversible steps in glycolysis (don’t reinvent the wheel when you don’t need to).
- Pyruvate can be converted to oxaloacetate (OAA) via enzyme pyruvate carboxylase and using 1 ATP.
- As a side note: amino acids can be converted to OAA and enter the pathway here.
- Then we can use GTP and enzyme PEPcarboxylase to convert OAA to phosphoenol pyruvate (at which point we can join the reversible steps in glycolysis).
Roadblock 2. How can we get from fructose 1,6-bisphosphate to fructose 6-phosphate?
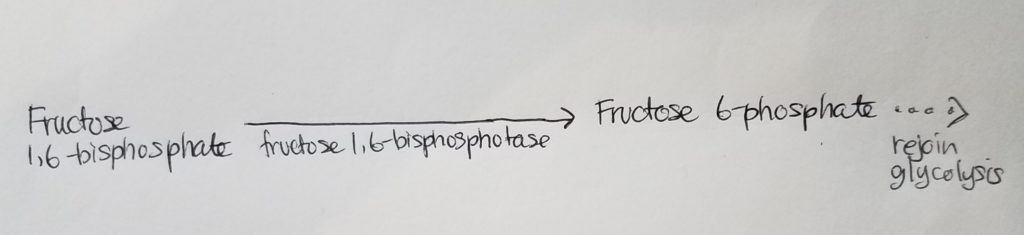
- We can use enzyme fructose 1,6-bisphosphotase to work-around this roadblock (irreversible reaction in glycolysis) to form fructose 6-phosphate. From fructose 6-phosphate, we can use the reversible steps of glycolysis. Again, don’t reinvent the wheel!
Roadblock 3. How can we get from glucose 6-phosphate back to glucose?
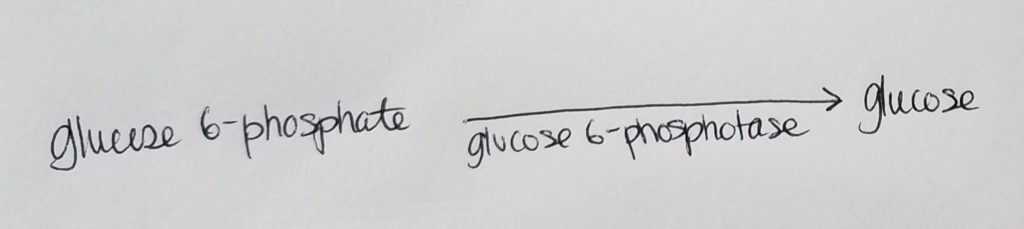
- No problem…we just wanna get back to good ole regular glucose!!
- We use this slick enzyme glucose 6-phosphotase to convert glucose 6-phosphate back to glucose.
Cori Cycle (aka Lactic Acid Cycle).
- In the muscle, glucose can make pyruvate which can be converted to lactate which can enter the bloodstream, which can enter the liver to be converted to pyruvate and back to glucose.
- Glycolysis happens in the muscles.
- Gluconeogenesis happens in the liver.
- When oxygen levels are low, anaerobic glycolysis happens.
- We need to regenerate NAD+.
- Side note: the heart muscle can also use lactate as an energy source.
Resources.
- Acetyl CoA, https://library.med.utah.edu/NetBiochem/FattyAcids/2_4.html
- Acetyl CoA, https://www.humpath.com/spip.php?article3611
- Major metabolic pathways, http://homepage.ufp.pt/pedros/bq/integration.htm
- Major metabolic pathways, http://opencourse.ncyu.edu.tw/ncyu/file.php/23/Central_metabolic_pathways_II.pdf
- Major metabolic pathways, https://www.sciencedirect.com/science/article/pii/S1097276510006672
- TCA, https://www.khanacademy.org/science/biology/cellular-respiration-and-fermentation/pyruvate-oxidation-and-the-citric-acid-cycle/a/the-citric-acid-cycle
- TCA, http://www.bioinfo.org.cn/book/biochemistry/chapt15/sim2.htm
- TCA, http://equilibrator.weizmann.ac.il/static/classic_rxns/classic_reactions/tca.html
- ETC, https://www.khanacademy.org/science/biology/cellular-respiration-and-fermentation/oxidative-phosphorylation/a/oxidative-phosphorylation-etc
- ETC, https://courses.lumenlearning.com/wm-biology1/chapter/reading-electron-transport-chain/
- ETC, https://www.unm.edu/~lkravitz/Exercise%20Phys/ETCstory.html
- ETC, https://www.cureffi.org/2013/11/08/biochemistry-08-the-citric-acid-cycle-and-the-electron-transport-chain/
- PDC, https://www.khanacademy.org/test-prep/mcat/biomolecules/krebs-citric-acid-cycle-and-oxidative-phosphorylation/v/regulation-of-pyruvate-dehydrogenase
- Pyruvate dehydrogenase, http://watcut.uwaterloo.ca/webnotes/Metabolism/TCAcycle.html
- ETC, http://watcut.uwaterloo.ca/webnotes/Metabolism/RespiratoryChain.html
- Gluconeogenesis, http://watcut.uwaterloo.ca/webnotes/Metabolism/Gluconeogenesis.html
- Glycolysis, http://watcut.uwaterloo.ca/webnotes/Metabolism/Glycolysis.html
- ETC, https://www.khanacademy.org/test-prep/mcat/biomolecules/krebs-citric-acid-cycle-and-oxidative-phosphorylation/v/electron-transport-chain
- Glycolysis, https://www.khanacademy.org/science/biology/cellular-respiration-and-fermentation/glycolysis/v/glycolysis-overview
- Glycolysis, https://www.khanacademy.org/science/biology/cellular-respiration-and-fermentation/glycolysis/a/glycolysis
- Glycolysis, http://watcut.uwaterloo.ca/webnotes/Metabolism/Glycolysis.html
- Glycolysis, https://courses.lumenlearning.com/boundless-biology/chapter/glycolysis/
- Pyruvate, https://courses.lumenlearning.com/wm-biology1/chapter/reading-pyruvate-oxidation/
- Pyruvate, https://www.chem.purdue.edu/courses/chm333/images/Fermentation%20Pyruvate.pdf
- Metabolic states, https://opentextbc.ca/anatomyandphysiology/chapter/24-5-metabolic-states-of-the-body/
- Metabolic states, https://courses.lumenlearning.com/suny-ap2/chapter/metabolic-states-of-the-body/
- Gluconeogenesis, https://www.khanacademy.org/test-prep/mcat/biomolecules/carbohydrate-metabolism/v/gluconeogenesis
References.
Bean, J. (2019). ETC & oxidative phosphorylation.
Bean, J. (2019). Glycolysis and gluconeogenesis.
Bean, J. (2019). TCA cycle.
Ferrier, D. (2017). Biochemistry (7th ed.). Philidelphia, PA: Lippincott Illustrated Reviews.
Lieberman, M., & Peet, A. (2017). Marks’ basic medical biochemistry: A clinical approach(5th ed.). Philadelphia, PA: LWW.