Last edited: 08.24 .2019
Outline.
- Fatty Acid Synthesis
- Cholesterol Metabolism
- Fed and Fasting States
Fatty Acid Synthesis.
- Fatty acid (FA) synthesis has quite the different pathway from fatty acid catabolism/breakdown.
- FA synthesis is made from glucose mainly in the liver (mostly liver) and adipocytes and mammary glands during lactation.
- Glucose is converted to pyruvate in the cytosol. Pyruvate enter the mitochondrial matrix and is converted to acetyl CoA and oxaloacetate.
- Acetyl CoA + oxaloacetate + H2O –> citrate (in mitochondria)
- Citrate is moved out to the cytosol.
- In the cytosol, citrate is cleaved into acetyl CoA and oxaloacetate.
- The acetyl CoA (in cytosol now) transforms —acetyl CoA carboxylase–> malonyl CoA.
- Malonyl CoA is the activated form of acetyl CoA.
- Malonyl CoA (gets decarboxylated) is used to grow the FA chain.
- When glucose is abundant and in excess, glucose may be converted and used this way for FA synthesis.
- The fatty acid synthase complex is located in the cytosol (uses cytosolic acetyl CoA).
- Citrate-Malate-Pyruvate shuttle provides cytosolic acetate units and reducing equivalens for the FA synthesis process.
- 1 NADPH is made for each acetyl CoA that’s transferred from the mitochondria to the cytosol via malic enzyme.
- Other NADPH molecules are produced from the pentose phosphate pathway as well.
Characteristics | Synthesis | Degradation |
---|---|---|
Location | Cytosol | Mitochondrial Matrix |
Activated Intermediates | Bound to ACP | Thioester of CoA |
Intermediates | -SH of acyl carrier proteins | -SH of CoA |
Activated Enzymes | FAS (multienzyme complex) | 4 distinct enzymes |
Substrte | Acetyl CoA | Fatty Acids |
Direction | Starts at methyl end | Starts at carboxyl end |
Cofactors | NADPH/NADP+ | FADH2/NADH, FAD/NAD+ |
Major Sites | Liver | Muscle & Liver |
Hormonal Regulation | High Insulin/Glucagon Ratio | Low Insulin/Glucagon Ratio |
Activator | Citrate | Free FA |
Inhibitor | FA CoA | Malonyl CoA |
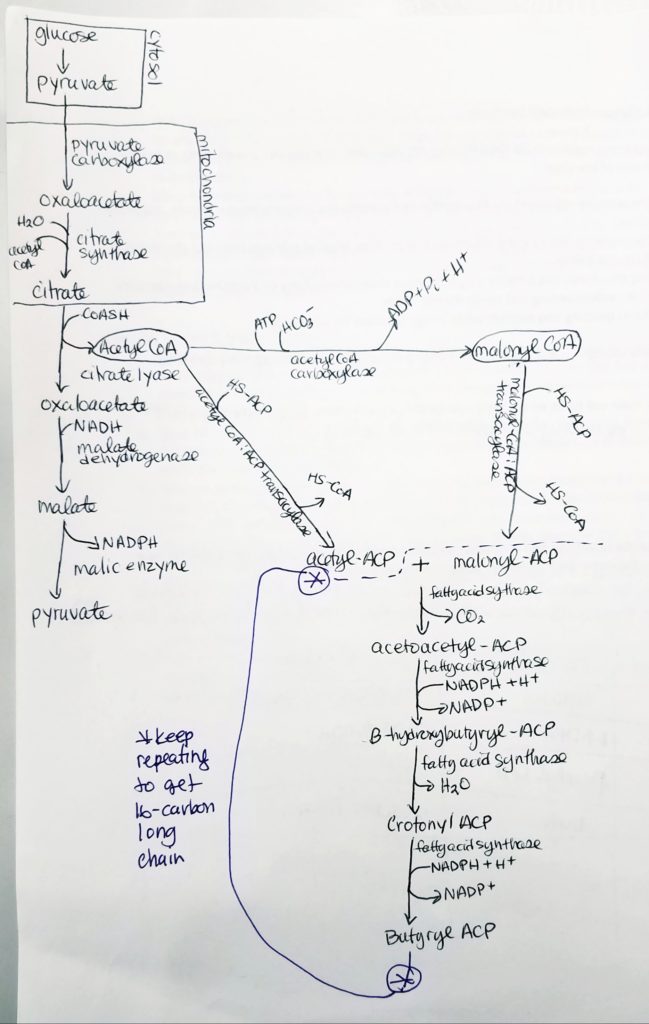
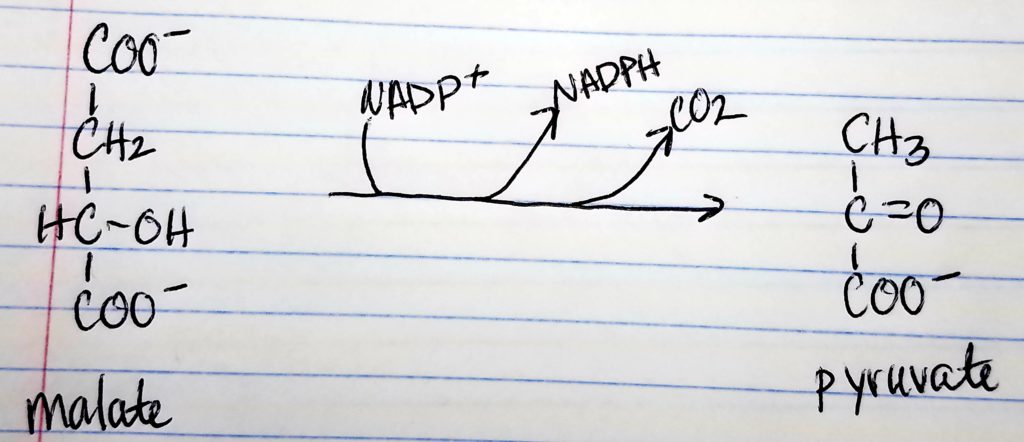
Acetyl CoA to Malonyl CoA.
- Acetyl CoA gets converted to Malonyl CoA via enzyme Acetyl CoA Carboxylase + coenzyme Biotin.
- This conversion is the most regulated step and it is the rate limiting step. It is reversible but this step is a commitment step to this pathway to make FAs.
- Note that not ALL our acetyl CoA is going down this path. Assume there’s plenty other acetyl CoA to make Acetyl ACP as well.
- 1 ATP is spent.
- One HCO3- (or CO2) is spent.
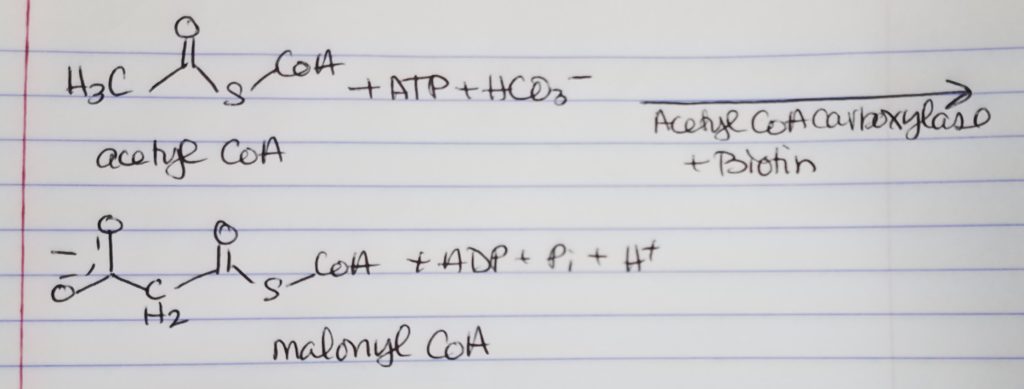
Acyl Carrier Protein (ACP).
- It is a complex with several binding sites for different activities.
- It is a dimer.
- The acyl carrier protein has a phosphopantetheine prosthetic group (can form thioester bonds) and a coenzyme A group.
- Prosthetic group: A non protein that combines with a protein/enzyme.
- The acetyl CoA and malonyl CoA get attached to ACP’s prosthetic group via the condensing enzyme acylmalonyl-ACP-condensing enzyme.
- The phosphopantetheine group (B5) is the “docking” location.
- The CoA part is where new materials are received.
Step 0: Activation/Loading.
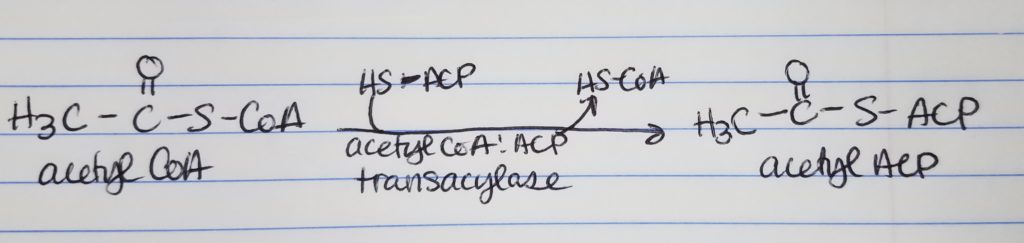
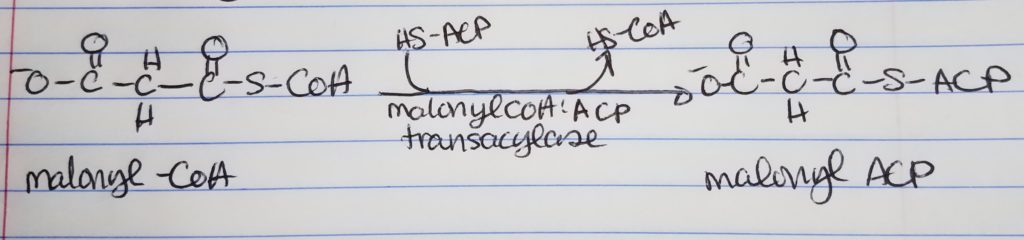
Fatty Acid Synthesis Step 1. Condensation.
- Condensation
- We are taking the “omega end” (the last end part) of acetyl ACP which is the H3C-C=O (keeping that from the acetyl ACP) and we get rid of the -O-C=O part of the malonyl ACP.
- Loading.
- Move the 2-carbon unit from malonyl-ACP to acetyl-ACP. Forms a 2-carbon keto-aceyl-ACP.
- Omega carbon = last carbon in a chain.
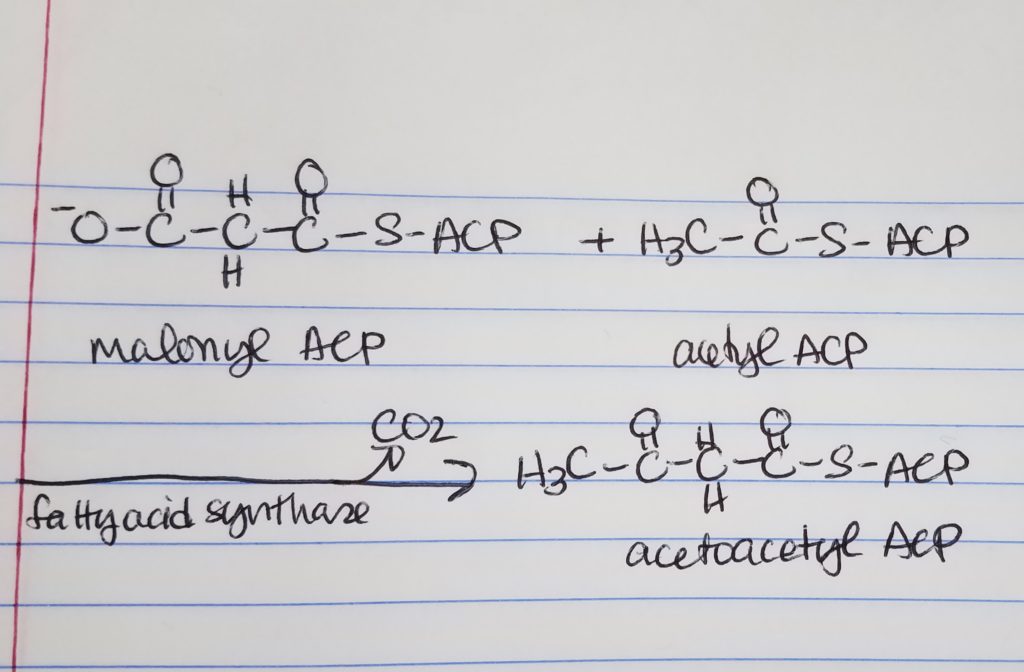
Fatty Acid Synthesis Step 2. Reduction.
- Reduction
- Get rid of the double bond, carbonyl, left of the CH2 group.
- Convert keto-acyl-ACP to hydroxyl-ACP.
- Spends 1 NADPH.
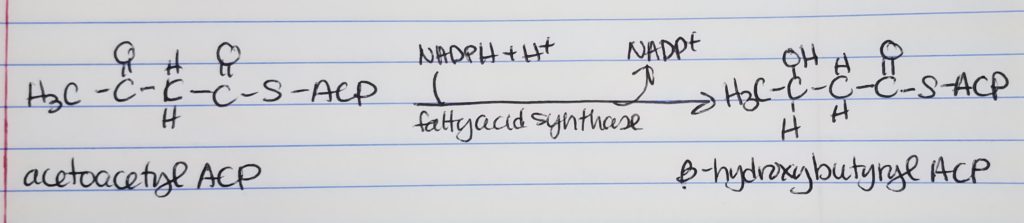
Fatty Acid Synthesis Step 3. Dehydration.
- Dehydration
- Want to get rid of the hydroxyl OH group by making a double bond.
- Also, get rid of the OH group, water is leaving group.
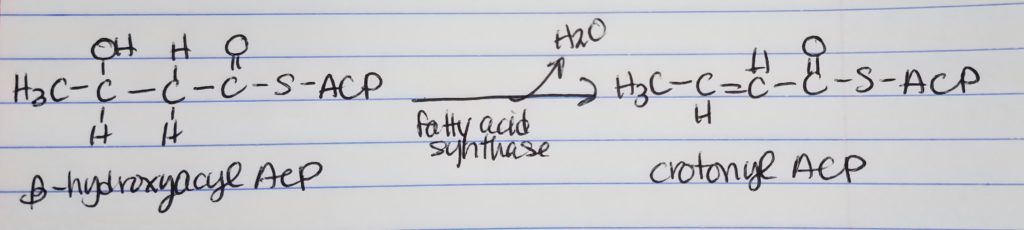
Fatty Acid Synthesis Step 4. Reduction.
- Reduction
- Want to reduce to get rid of that double bond (trans H), left of the carbonyl.
- Spend 1 NADPH.
- With butyrl ACP, that is a 4-carbon chain with 2-carbons from the acetyl ACP.
- Hereafter, the cycle is repeated by adding malonyl ACP. This gives an addition of 2 more carbons to the chain.
- 1st time through the cycle, 2-carbons from acetyl ACP and 2-carbons from malonyl ACP = 4-carbon chain.
- 2nd time through the cycle, add 2 more carbons from malonyl to get a 6-carbon chain.
- 3rd time through the cycle, add 2 more carbons from malonyl to get a 8-carbon chain.
- 4th time through the cycle, add 2 more carbons from malonyl to get a 10-carbon chain.
- 5th time through the cycle, add 2 more carbons from malonyl to get a 12-carbon chain.
- 6th time through the cycle, add 2 more carbons from malonyl to get a 14-carbon chain.
- 7th time through the cycle, add 2 more carbons from malonyl to get a 16-carbon chain.
- STOP at 16-carbon chain which is Palmitate because fatty acid synthase cannot handle anything more than the 16-carbon chain.
- 7 cycles are needed to make the 16-carbon palmitate.
- 7 cycles >> 1 molecule of acetyl CoA and 6 molecules of malonyl CoA; 14 NADPH; 7 ATP.
- To make a complete palmitate:
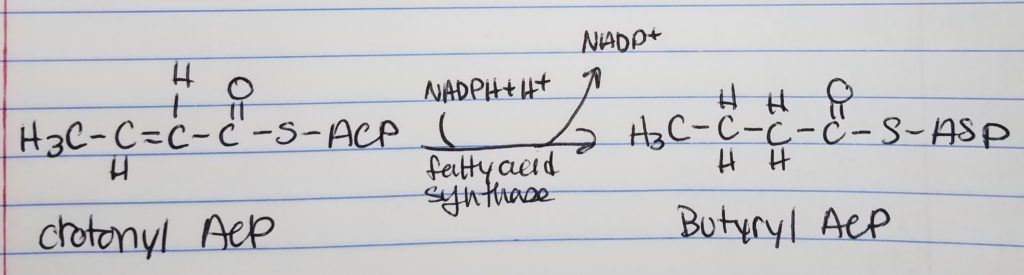
How to Make a Long Fatty Acid? Keep repeating…
- Keep adding malonyl ACP (adds 2 carbons each time) until a 16-carbon chain is reached.
- Fatty acid synthase’s limit is 16 carbon chain.
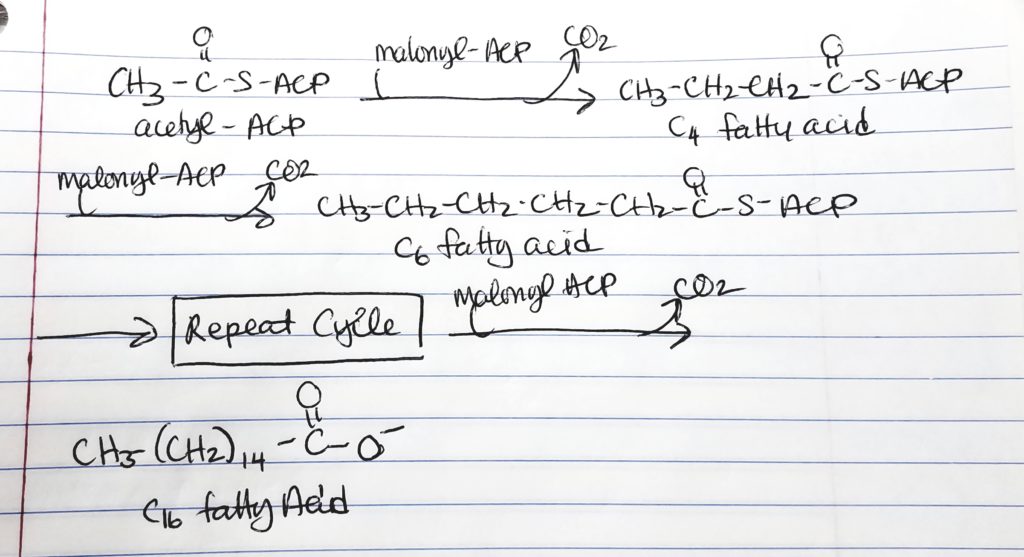
Palmitate.
- At 16-carbon long FA chain, the enzyme thioesterase hydrolyzes the FA acyl group in order to make “free” palmitate.
- palmitoyl-ACP + H2O –thioesterase–> palmitate + ACP-SH
- Then palmitate can undergo elongation or unsaturation to make FAs.
Elongation and Unsaturation.
- Elongase is an enzyme in the smooth endoplasmic reticulum (ER) that adds 2-carbons onto a FA chain via malonyl CoA.
- Desaturase is an enzyme that adds cis double bonds up to position delta-9 in the ER in mammals. Humans can only use cis double bonds and not trans.
Summary of Key Points of FA Synthesis Steps.
- Step 0. Activation-Loading. Acetyl CoA and malonyl CoA need to be “loaded” on to the Acyl Carrier Protein (ACP). Enzymes: acetyl-CoA:ACP transacylase; malonyl-CoA:ACP transacylase. The original CoA’s are replaced by ACP designation.
- Step 1. Condensation. Acetyl-C0A:ACP and malonyl-CoA:ACP join to form a 4-carbon chain via fatty acid synthase. Enzyme: fatty acid synthase. A 2-carbon keto-acyl-ACP is formed. Released: CO3.
- Step 2. Reduction. Convert the carbonyl carbon adjacent to the omega carbon from a double bonded to O to a single bond OH group. Converts keto-acyl-ACP to hydroxyacyl-ACP. Enzyme: fatty acid synthase. Spends: NADPH + H+ to NADP+.
- Step 3. Dehydration. Need to remove the hydroxyl group at the carbon adjacent to the omega carbon. Forms a trans double bond between the second and third “to last” carbons in the chain. An enoyl is formed. Enzyme: fatty acid synthase. Released: H2O.
- Step 4. Reduction. Get rid of the double trans bond from step 3 via reduction to form a fully saturated 4-carbon chain. Enzyme: fatty acid synthase. Spends: NADPH + H+ to NADP+.
Release Palmitate.
- Fatty acid synthase can make up to a 16 carbon chain.
- Palmitate is a 16 carbon chain.
- When the cycle repeats and makes a 16-carbon long chain, thioesterase hydrolyzes it and frees up palmitate.
- Palmitate itself can then elongate or desaturate (convert double bonds to single bonds).
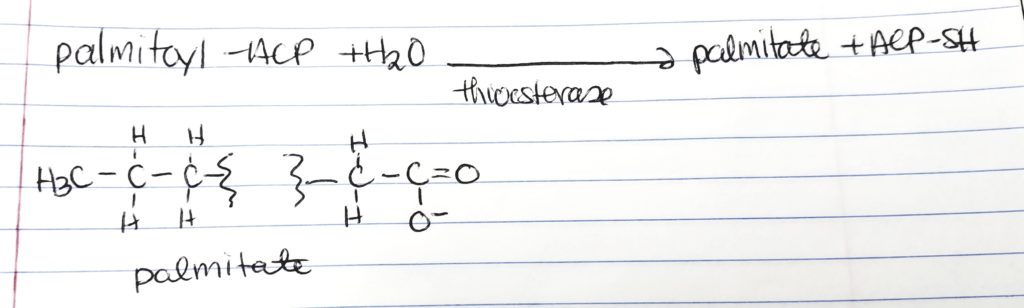
Elongation an Desaturation.
- After 16 carbons, elongase takes over. Elongase functions very much like FA synthase.
Regulation of Fatty Acid Synthesis.
- Acetyl CoA carboxylase (ACC) is controlled by: glucagon, epinephrine, insulin.
- ACC & glucagon. If glucagon levels are high, that means the body needs more blood sugar. It’s in a fasting state indicating that it is NOT the right time to be making fatty acids. Glucagon is an inhibitor.
- ACC & epinephrine. Increased levels of epinephrine inhibit ACC. Increased levels of epinephrine indicate that the body needs more blood sugar (fight or flight). That is NOT the right time to make FAs.
- ACC & insulin. Increased levels of insulin indicate that the blood has too much sugar floating around and insulin tells the cells to let blood sugar in (store or use). Insulin stimulates ACC which stimulates the production of FAs.
- Other regulation types: citrate, palmitoyl CoA, AMP.
- ACC is controlled via phosphorylation.
- Insulin –> stimulates FA –> causes dephosphorylation of ACC.
- Glucagon/epinephrine –> inhibits FA –> causes phosphorylation of ACC.
- Protein kinase (AMP-PK): activated by AMP; inhibited by ATP.
- ACC is inactivated when ATP is low.
- Citrate allosterically activates ACC.
- Citrate levels are high when acetyl CoA and ATP levels are high.
- Isocitrate dehydrogenase is inhibited by ATP.
- Carboxylase is allosterically inhibited by palmitoyl CoA.
- Global regulation: +insulin, -glucagon, -epinephrine.
- Local regulation: +citrate, -palmitoyl CoA, -AMP.
- Formation of malonyl CoA inhibits carnitine acyltransferase I
Global Regulation.
- If energy is low, then it is not the right time to make fatty acids.
- ACC is inactivated when ATP levels are low.
- Global regulations is achieved via reversible phosphorylation.
- Aceytyl CoA is inhibited by phosphorylation and stimulated by dephosphorylation.
- Insulin stimulates FA synthesis via dephoshorylation of ACC.
- Glucagon and epinephrine inhibit ACC via phosphorylation.
- Protein kinase is stimulated by AMP and inhibited by ATP.
Local Regulation.
- Citrate allosterically activates acetyl CoA.
- Citrate levels are high when acetyl CoA and ATP levels are in excess (isocitrate inhibited by ATP).
- Palmitoyl CoA allosterically inhibits carboxylase.
Fed.
- Increased insulin levels.
- Inhibit hydrolysis of triacylglycerides.
- Stimulates increase of malonyl CoA.
- Increased malonyl CoA inhibits carnitine acyltransferase I.
- FA stay in cytosol (FA oxidation enzymes are in mitochondria); so FA do not oxidize.
Fasting.
- Epinephrine and glucagon at increased levels.
- They stimulate adipose cell lipase.
- Levels of free FA increase.
- Inhibit ACC to decrease formation of malonyl CoA (causes more FA transported to mitochondria for beta-oxidation).
Cholesterol Metabolism.
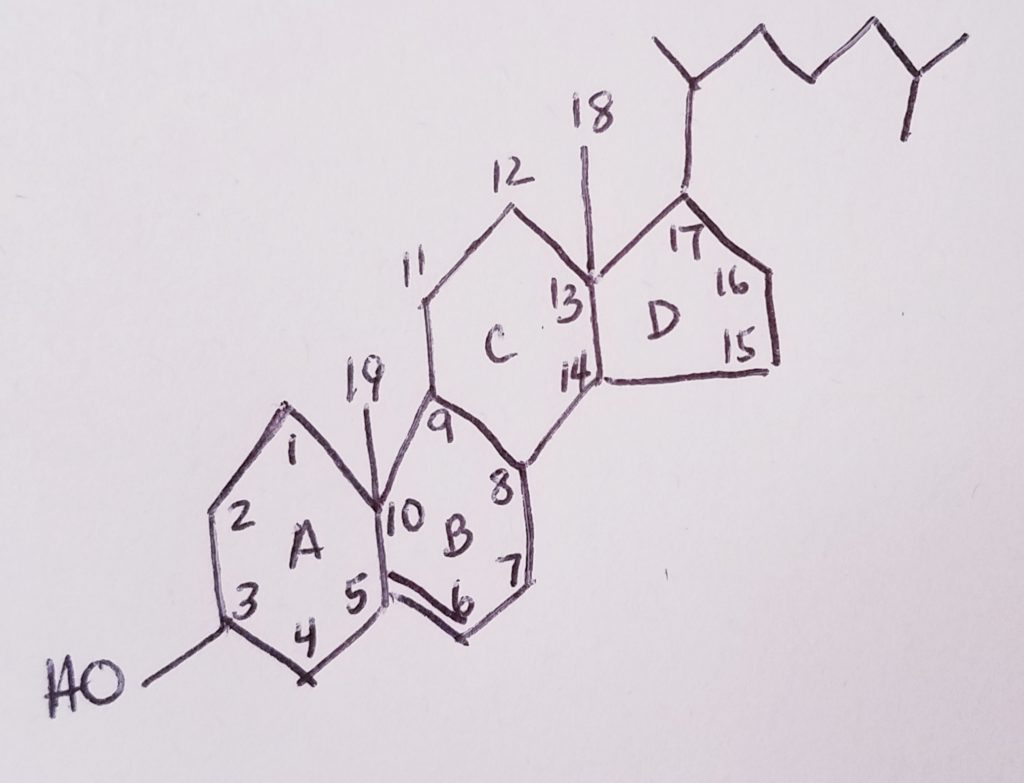
- Cholesterol = steroid nucleus (sterane) that contains lipid and has a hydrocarbon tail.
- Cholesterol is a lipid.
- 4-ring steroid nucleus. It’s very hydrophobic.
- Ring A is a hexagon with OH at carbon-3 position.
- Ring B is a hexagon with a CH3 (methyl) at position carbon-10. The methyl’s carbon is #19.
- Ring C is a hexagon with a CH3 (methyl) at position carbon-12. The methyl’s carbon is #18.
- The hydrocarbon tail would be at carbon-17.
- A double bond from carbon-5 to carbon-6
- 27-carbon non-glyceride lipid.
- Amphipathic: polar OH at C3; non-polar nucleus and tail at C17.
- Made by most body cells: liver, intestines, cortex, brain, reproductive tissues.
- Plasma cholesterol is often esterified (cholesterol ester at C3).
Phytosterol.
- Plants don’t use cholesterol. Mammals only make cholesterol.
- The plant-equivalent of cholesterol is phyosterol.
Flow of Major Cholesterol Intermediates: Number of Carbons and Number of Acetyl CoA.
Stage 1. Acetyl CoA to Mevalonate (2-carbons to 6-carbons).
- 3 x acetyl CoA (2-carbon) –> mevalonate (6-carbon).
- Enzymes: thiolase, HMG-CoA synthase, HMG-CoA reductase.
- Cost: 2 NADPH.
- Leaving: 3x CoA-SH.
- Thiolase to cleave out the thiol and CoA groups. Also joining the first two acetyl CoA’s.
- CoA-SH is leaving group.
- B-Hydroxy-B-methylglutaryl-CoA Synthase (HMG-CoA synthase) to join the third acetyl CoA group and another CoA-SH leaves.
- HMG-CoA reductase uses 2 NADPH to get rid of the last CoA-SH group.
- B-Hydroxy-B-methylglutaryl-CoA is the rate-limiting step.
Stage 2. Mevalonate to Isopentenyl Pyrophosphate (IPP) (6-carbons, -1, 5-carbons).
- Enzymes: mevalonate-5-phosphotransferase, phosphomevalonate kinase, kinase, pyrophosphomevalonate decarboxylase, isopentenyl pyrophosphate isomerase.
- Cost: 3 ATP.
- Leaving: CO2, Pi.
- The CO2 is the -1 carbon from the mevalonate.
Stage 3A. IPP to Farnesyl Pyrophosphate.
- Need 6x mevalonate (5 carbon chain, needed 3 acetyl CoA) to make squalene (30 carbon chain, 18 acetyl CoA)
- Enzymes: cis-prenyl transferase.
- Cost:
- Leaving: 2 PPi.
- The end of stage 3A gives us a 15 carbon long chain (or 3 isoprenes) farnesyl pyrophosphate.
Stage 3B. Farnesyl Pyrophosphate to Squalene.
- Enzymes: squalene synthase.
- Cost: NADPH + H+.
- Leaving: 2 PPi.
Stage 4. Squalene to Lanosterol (both are 30 carbons long).
- Enzymes: squalene monooxygenase, cyclase.
- Cost: NADPH + H+, O2.
- Leaving: NADP+, H2O.
- This step is important to close the ring.
Stage 5. Lanosterol to Cholesterol (30 carbons to 27 carbons).
- Multistep.
Hydrolyzable | Non-Hydrolyzable |
---|---|
Esters | Hydrocarbons |
Fats | Carotenoids |
Waxes | Alcohols |
Sterol Esters | Sterols |
Phospholipids | Steroids |
Phosphatidates | Acids |
Phosphtids | Fatty Acids |
Spingolipids | Eicosanoids |
Glycolipids |
Regulation of HMG-CoA Reductase.
- This is the rate-limiting enzyme to make cholesterol and other isoprenoids.
- It is a transmembrane (a type of integral membrane protein that spans the membrane) protein.
- It is anchored in the endoplasmic reticulum membrane.
- It has 8 domains.
- It is the target of many statin drugs (lipid lowering).
- Inihibits production of cholesterol in liver.
- Phosphorylated form: Inactive.
- DePhosphorylated form: Active.
- Insulin/thyroxine stimulates HMG-CoA reductase>>dephosphorylate HMG-CoA.
- Glucagon/cortisol inhibits HMG-CoA reductase>>phosphorylate HMG-CoA.
- If there’s high cellular cholesterol, the gene to make more HMG-CoA reductase enzyme is suppressed. This results in fewer and fewer copies of the HMG-CoA reductase enzyme and hence, the production of cholesterol slows down.
Statins, Muscle Pain, Ubiquinone (CoQ).
- It is unknown why statins may cause muscle pain in some people.
- CoQ supplementation may be helpful and CoQ is relatively “safe”.
Cholesterol Esterification.
- Cholesterol may “hitch a ride with” lipoproteins, but that limits cholesterol to the surface or lipid portion of those lipoproteins.
- Esterifying cholesterols allow them to become more incorporated or at least, not limited to reside “on the surface”.
- Esterification allows cholesterols more access to the interiors of lipoproteins or other structures that have both the hydrophobic and hydrophillic characteristics.
- Esterification also allows for greater carrying capacity of lipoproteins.
- Esterification converts cholesterol to an even more hydrophobic form.
- This is done in the liver via enzyme acyl-CoA-cholesterol acyl transferase (ACAT).
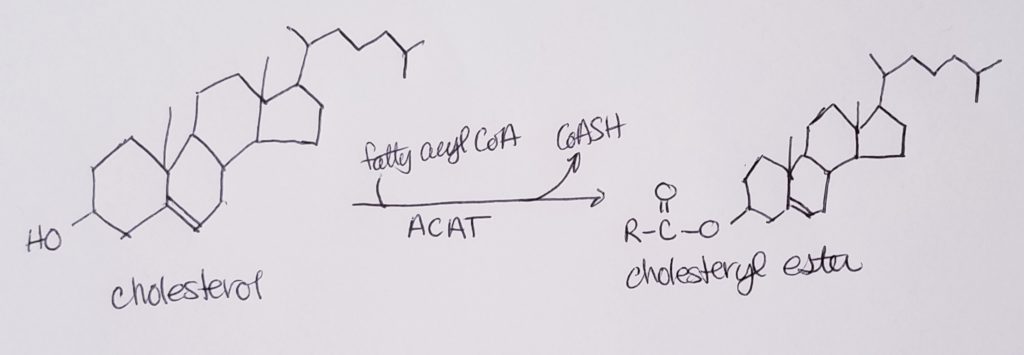
Bile Acids and Bile Salts.
- Bile is a mix of: water, electrolytes, organic materials, cholesterol, lipids, phospholipids, bilirubin and other waste products that get secreted into the bile and excreted.
- Bile helps us breakdown food.
- Bile helps us absorb fat soluble vitamins A, D, E, K.
- Adults can make 400-800 ml of bile/day.
- Liver cells (hepatocytes) make bile which run down the canaliculi ducts to the bile ducts. During this journey, bicarb is added into the mixture.
- Bile ends up in the gall bladder which helps to concentrate the mixture (during the body’s fasting state). Bile can be 5x more concentrated.
- To get rid of excess cholesterol, the bile is the way to go. The bile helps make cholesterol less hydrophobic via bile acids and lipids (e.g. lecithin). Cholesterol gets trapped in all the muck and can excrete it.
- Bile acids/salts can emulsify fats and take on a micelle form.
- Cholesterol can be converted into bile cholic (2nd most abundant) and chenodeoxycholic (most abundant) acids.
- Cholic and chenodeoxycholic acids can be conjugated to glycine or taurine. This would make the “active forms”.
- Primary bile acids made in liver via cholesterol made in the liver: cholic and chenodeoxycholic acids.
- These primary bile acids travel into the small intestine where bacteria reacts with the primary acids. They undergo dehydroxylation (a heating reaction where OH is released as water). The result are the secondary bile acids.
- Secondary bile acids: Deoxy Cholic Acid (Deoxy CA or DCA) and Litho Chenodeoxy Acid (Litho CA or LCA)
- To make bile acids…the rate determining step is:
Cholesterol –7alpha-hydrolase–>7alpha-hydroxycholesterol. - 7alpha-hydroxycholesterol is the precursor to bile acids.
- The glycine or taurine can be found attached onto the 24th carbon of the hydrocarbon tail.
- Bile salts glycocholic acid and taurocholic acid.
- Bile salts help digestion, eliminate waste products, help absorb fat-soluble vitamins and other components that the body needs.
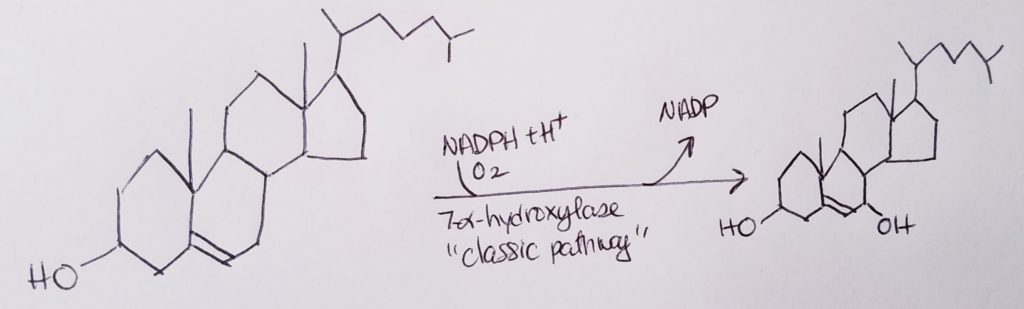
Vitamin D, Calcitriol.
- Vitamin D3 (cholecalciferol) is made in the skin in the presence of sunlight.
- 25-hydroxycholecalciferol is made from cholecalciferol
- In the liver, Cholecalciferol –25-hydroxylase–> 25-hydroxycholecalciferol
- In the kidney with enzyme 1-alpha-hydroxylase helps convert 25-hydroxycholecalciferol into the active form of vitamin D, 1,25-dihydroxycholecalciferol.
- Sources of natural cholecalciferol (vit D3): fish and meat.
- Sources of ergocalciferol (vit D2): plants and fungi.
5 Types of Steroid Hormones Derived from Cholesterol.
- Mineralocortictoids. Made in adrenal cortex. Eg. Aldosterone C21 (conservation of Na+).
- Glucocorticoids. Made in almost every mammalian cell. Eg. cortisone C21.
- Androgens. Male testes. Eg. testosterone C19.
- Estrogens. Ovaries. Eg. Estradiol
- Progesterone. Ovaries, placenta, adrenal gland.
Lipoproteins (transport cholesterol).
- Lipoproteins are proteins that can help transport lipids.
- Types of lipids that may be transported via lipoprotein: fatty acids, triacylglycerols, phospholipids, free cholesterol, cholesterol ester, fat-soluble vitamins.
- 5 main types of lipoproteins:
1.Chylomicron. Transport dietary lipids from intestines to other places in the body.
2. VLDL, very low density lipoprotein. Carry cholesterol to tissues.
3. IDL, intermediate density lipoprotein. Functions like LDL, carrying cholesterol and fats.
4. LDL, low density lipoprotein. Can buildup in vessels.
5. HDL, high density lipoprotein. Carry cholesterol back to liver. - Density and size are inversely proportional (denser, smaller vs. less dense, fluffier and bigger).
- Liver: makes VLDL and HDL.
- Intestine: chylomicron, HDL.
Apolipoproteins (bind lipids to proteins to make lipoprotein).
- Apo A1. HDL.
- Apo B48. Chylomicron.
- Apo B100. VLDL, IDL, LDL.
- Apo C2. VLDL, chylomicron.
- Apo E. IDL, chylomicron remnants.
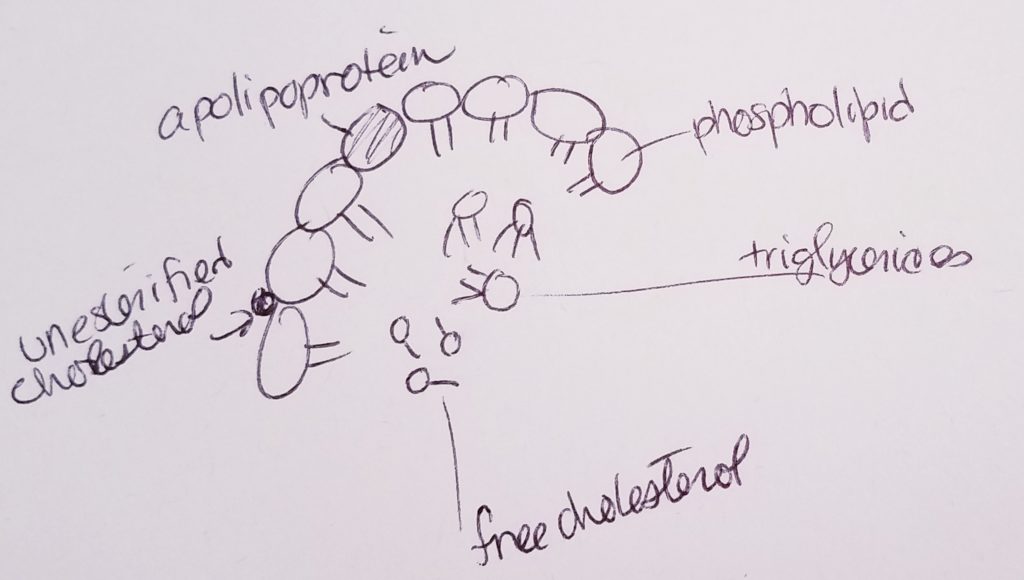
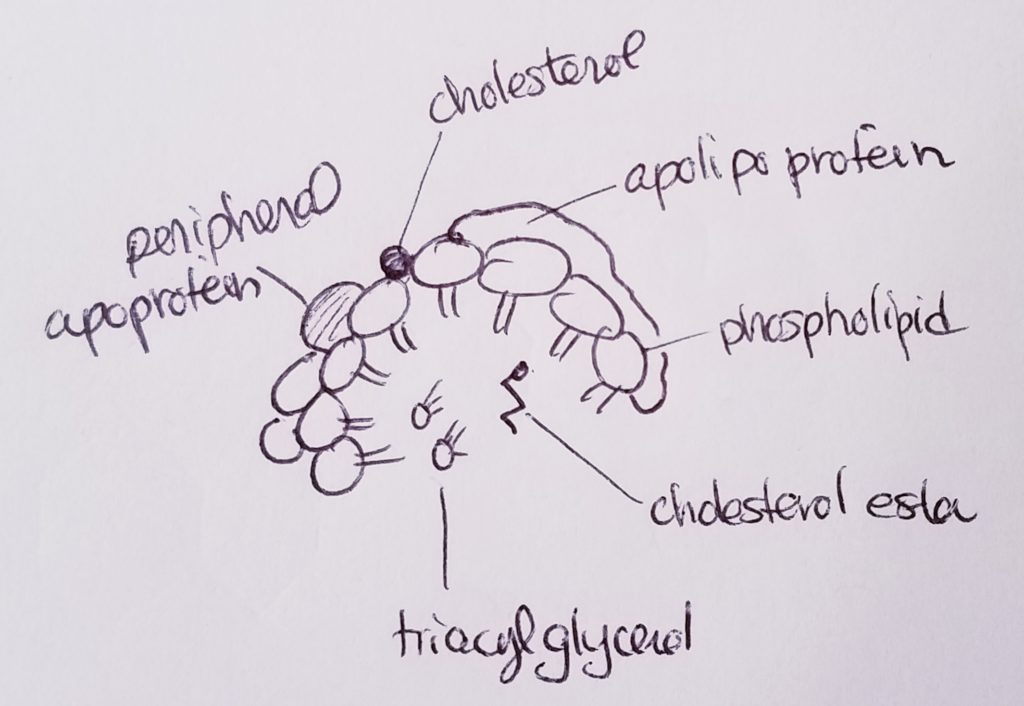
Hormones.
- Hormones are chemical messengers (from the endocrine system) that help regulate the body and maintain homeostasis.
- Some hormones travel all over the body via bloodstream.
- Some hormones are more localized.
Fed & Fasting States.
- Hormones are chemical messengers. Released from the endocrine glands, they get secreted into blood.
- Pancreas > Islet of Langerhans (beta cells) makes insulin.
- Pancreas > Alpha cells make glucagon.
- Pancreas > Delta cells make somatostatin.
- Note somatostatin: It’s made in more than one place. In the pancreas, somatostatin can inhibit insulin and glucagon. Somatostatin that’s produced in the pancreas affects/regulates the pancreatic hormones.
Insulin.
- Insulin dominates the FED state.
- Stimulates: glucose oxidation; glycogen synthesis; fat synthesis; protein synthesis.
- Insulin is a polypeptide derived from the prohormone proinsulin.
- The C-peptide is cleaved from the insulin precursor.
- Insulin has two chains connected via disulfide bridges.
- Insulin stimulates tissues (esp. liver, muscle, adipose) to increase the uptake of glucose and amino acids. If the glucose/amino acids isn’t needed for immediate use, then package it into a storage form.
- Insulin stimulates glycogen formation (for stored energy).
- Insulin increases FA synthesis in liver.
- Stimulates potassium uptake.
- Inhibits glucagon production.
Regulators of Insulin.
- +Blood glucose. When blood glucose is abundant, insulin is activated to tell cells to use/store the blood glucose.
- +Amino acids.
- +Neural input.
- +Gut hormones.
- -Epinephrine (adrenergic).
Regulators of Glucagon.
- -Glucose.
- -Insulin.
- -Amino acids.
- +Cortisol.
- +Neural (stress).
- +Epinephrine.
Other Regulators.
- Glucocorticoids (cortisol): stimulate gluconeogenesis and lipolysis and increase protein breakdown.
- Epinephrine/norepinephrine: stimulate glycogenolysis and lipolysis (exercise).
- Growth Hormone: stimulates glycogenolysis and lipolysis.
Thought Exercise: What Happens Right After a Meal vs. What Happens a Few Hours After a Meal??
Digestion.
- Digestion is the process of converting and breaking down food into simpler components that the body can absorb (and deal with) and use.
- The digestive/GI tract is where digestion-absorption takes place.
- The route is: mouth, esophagus, stomach, small intestine, large intestine.
- Denaturing: is unfolding of proteins or altering the structure in 3D space without breaking bonds.
- Breaking down: is snipping or cutting protein via break bonds.
Digestive Path.
- Salivary glands. Start salivating to help lubricate foodstuffs.
- Mouth. Amylase helps start the breakdown of carbs. Mechanical and chemical breakdown starts.
- Pharynx. Swallows foods/liquids.
- Esophagus. Transports food.
- Stomach. Churns foods. Pepsin helps break down protein. Stomach HCl helps breakdown food and kill germs. Mucosal lining helps protect the stomach and our tissues from the harsh acidity.
- Liver. Makes bile. Filters nutrients. Stores vitamins and iron. Destroys wastes and toxic materials.
- Gall Bladder. Stores and concentrates bile.
- Pancreas. Makes hormones. Bile ducts pass through to empty bile in duodenum of the small intestine. Hormones insulin and glucagon. Add bicarb to the bile and help neutralize stomach acid. Trypsin and chymotrypsin help digest proteins. Amylase helps digest polysaccharides. Lipase digests lipids.
- Small Intestine. Absorb most of our stuff.
- Large Intestine. Absorb most of our water.
- Rectum.
- Anus.
- Pancreatic amylase: helps break down dietary carbohydrates.
- Brush border disaccharidases: enzymes that help break down disaccharides in the small intestinal wall.
- Disaccharides : maltose (glucose x2); sucrose (glucose + fructose); lactose (glucose + galactose).
Resources.
- FA elongation & denaturation, https://youtu.be/eG08eClNoXA
- Fatty acid synthesis (Moof), https://youtu.be/w1cAMmXhJGs
- Fatty acid synthesis, part 1, https://www.khanacademy.org/test-prep/mcat/biomolecules/fat-and-protein-metabolism/v/fatty-acid-synthesis-part-i
- Fatty acid synthesis, https://www.khanacademy.org/test-prep/mcat/biomolecules/fat-and-protein-metabolism/v/fatty-acid-synthesis-part-ii
- Fatty acid synthesis, https://bio.libretexts.org/Bookshelves/Cell_and_Molecular_Biology/Book%3A_Cells_-_Molecules_and_Mechanisms_(Wong)/6%3A_Metabolism_II_%E2%80%93_Anabolic_Reactions/6.6%3A_Fatty_Acid_Synthesis
- Fatty acid synthesis, https://www.diapedia.org/metabolism-and-hormones/5105592814/fatty-acid-oxidation-and-synthesis
- Cholesterol, https://www.cdc.gov/cholesterol/index.htm
- Cholesterol, http://watcut.uwaterloo.ca/webnotes/Metabolism/Cholesterol.html
- Cholesterol, https://bio.libretexts.org/Bookshelves/Biochemistry/Book%3A_Biochemistry_Free_and_Easy_(Ahern_and_Rajagopal)/06%3A_Metabolism_I/6.08%3A_Cholesterol_Metabolism
- Cholesterol, https://youtu.be/3utYm3ouzaU
- Cholesterol, https://youtu.be/nurZ2nNovp8
- Cholesterol from the Biochem channel, https://youtu.be/seRkOCEfWpo
- HMG-CoA reductase, https://www.healio.com/cardiology/learn-the-heart/cardiology-review/topic-reviews/hmg-coa-reductase-inhibitors
- Cholesterol esterification, https://www.sigmaaldrich.com/technical-documents/articles/biofiles/cholesterol-esterification.html
- Bile, http://www.vivo.colostate.edu/hbooks/pathphys/digestion/liver/bile.html
- Cholic acid, https://en.wikipedia.org/wiki/Cholic_acid
- Chenodeoxy acid, https://en.wikipedia.org/wiki/Chenodeoxycholic_acid
- 7-alpha-hydroxycholesterol, https://themedicalbiochemistrypage.org/bileacids.php
- Vitamin D, http://www.vivo.colostate.edu/hbooks/pathphys/endocrine/otherendo/vitamind.html
- Lipoproteins, https://www.mabtech.com/knowledge-center/applied-research/apolipoproteins
- Insulin, https://www.yourhormones.info/hormones/insulin/
- Digestion, https://opentextbc.ca/anatomyandphysiology/chapter/23-7-chemical-digestion-and-absorption-a-closer-look/
References.
Bean, J. (2019). Cholesterol metabolism.
Bean, J. (2019). Fatty acid synthesis.
Bean, J. (2019). Fed and fasting states.
Ferrier, D. (2017). Biochemistry (7th ed.). Philidelphia, PA: Lippincott Illustrated Reviews.
Lieberman, M., & Peet, A. (2017). Marks’ basic medical biochemistry: A clinical approach(5th ed.). Philadelphia, PA: LWW.