Oxygen requirements vary per organism.
Aerobes. Need oxygen for growth. O2 is the final electron acceptor in their metabolic pathway. You may notice growth at the top of a broth tube or agar deep tube (after stab inoculation). If someone has an open wound, aerobes may be found on (or close to) the wound’s surface.
Microaerophiles. As the name suggests (“micro” small), these organisms need a little oxygen for their growth. Too much oxygen inhibits growth or kills these types of organisms. You may notice growth towards the middle of a broth tube or agar deep tube (after stab inoculation). If someone has an open wound, microaerophiles may be found a bit below the wound’s surface.
Obligate/strict anaerobes. These organisms growth without oxygen, and the presence of oxygen would be destructive (via build-up of the free radical superoxide). O2 is not the final electron acceptor. Obligate anaerobes lack the enzymes superoxide dismutase and catalase which function to breakdown superoxide to oxygen and water. You may observe growth at the bottom of a broth tube of agar deep tube when placed in a GasPak system. These organisms may reside/penetrate deep in a wound, away from the surface.
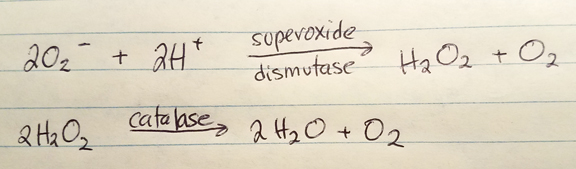
Aerotolerant anaerobes. These are fermentors and O2 isn’t the final electron acceptor. They do produce superoxide dismutase and catalase which help them “tolerate” an environment with oxygen. You may find growth below the surface of a broth tube or agar deep tube. These organisms may reside below a wound’s surface.
Facultative anaerobes. These organisms can grow with or without the presence of oxygen. Oxygen may be the final electron acceptor, but nitrate or sulfate compounds may be utilized in the absence of oxygen.
Clinical significance. It helps to narrow down the possibilities if one can determine the oxygen requirements of an unknown organism.
Cultivating anaerobes. There are several ways to cultivate anaerobes, but you can break these methods down into two groups: methods that require a sealed container (e.g. sealed jar), and methods that do not require a sealed container.
Methods using a sealed container. The container must be air-tight and there must be some way to get rid of any oxygen trapped inside the container.
Brewer jar. Uses a pump to evacuate the oxygen and replace it with 95% nitrogen and 5% carbon dioxide.
GasPak. No pump or evacuation is required. A foil package inside the jar generates hydrogen and carbon dioxide when water is added. In the lid, there’s a palladium catalyst to help hydrogen to combine with residual oxygen making the end-product, water. There’s usually a methylene blue indicator strip which is blue in the presence of oxygen and is colorless in the absence of oxygen.
Chromium-sulfuric acid. This requires the production of hydrogen via 15% H2SO4 with Cr+2. The hydrogen is evolved and O2 is evacuated.
Other methods for culturing anaerobes do not require the use of a sealed container.
Shake-culture (solid medium). While agar is in the liquid phase, it is inoculated with a loopful of organism. The tube is then shaken/agitated and rapidly cooled to a solid form, then incubated. Strict anaerobes are likely to grow at the bottom of the tube.
Pyrogallic acid (solid medium). Agar slants are inoculated. A cotton plug is placed at the upper portion of the tube (almost touching the top of the agar). Pyrogallic acid crystals are placed on top of the cotton plug, and sodium hydroxide is added. These act to pull oxygen out of the area below the cotton plug.
Paraffin plug (broth medium). A liquid reducing substance is used. Heat is applied to drive off the oxygen gas, cooled rapidly and inoculated. The tube is sealed with 1/2 inch of melted paraffin, then incubated.
Fluid thioglycollate (broth medium). Sodium thioglycollate binds to oxygen gas. A redox indicator (e.g. resazurin) may be used as a visual aid.
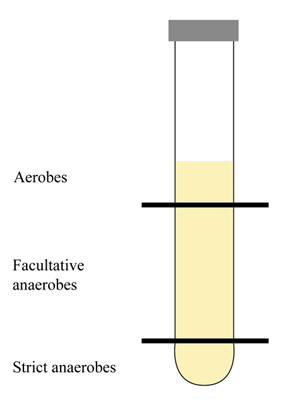
Reference
Cappuccino, J. G., & Welsh, C. (2018). Microbiology: A laboratory manual.